Abstract
Radiotherapy plays an important role in organ preservation for bladder cancer. Delivering radiation accurately can be challenging, in part because the bladder and surrounding organs may change position, volume, and shape between and during the fractions of a treatment course. This variability has been accounted for by increasing the margins around the treatment targets, which can expose more normal tissue to radiation, and increase the likelihood of normal tissue complications. An alternative strategy is to alter, or adapt, the radiotherapy treatment plan to account for such inter-fraction changes, a strategy termed ‘adaptive radiotherapy’ (ART). ART allows smaller target volumes to be treated, and may reduce complications. Approaches to ART include offline adaptation strategies and online strategies, which includes choosing a plan of the day (PoD) based on pre-treatment imaging and magnetic resonance (MR), or with cone-beam CT (CBCT)-guided daily plan re-optimisation. Here, the authors review these ART strategies and trials exploring the dosimetric and clinical benefits of ART relative to non-ART bladder radiotherapy.INTRODUCTION
Bladder cancer is the sixth most common cancer, with an estimated 82,290 new cases in 2023.1 Radiation therapy has many clinical applications for bladder cancer, including the opportunity to cure cancer with organ preservation as part of trimodality strategy for muscle-invasive disease, and in the palliative setting for symptomatic local disease.2,3 Radiotherapy has been used to treat patients with bladder cancer since the 1920s, with the first international consensus guidelines published in July 1983, following the First International Symposium of Tumors of the Urinary Bladder in Paris, France.4-6 In the past few decades, the techniques used to deliver radiation therapy for bladder cancer have evolved significantly to overcome challenges related to daily changes in the size, shape, and position of the bladder.7,8 For example, with conventional radiation, large margins were added to target volumes to account for the dynamic volume of the bladder throughout the course of treatment, which could result in higher local failure rates from geographic misses, and increased toxicities because of increased exposure of normal tissue.9 With the development of new technology, ART has emerged as a promising solution to improve target precision, with the potential to improve clinical outcomes. The aim of this review is to: review how advances in technology and treatment techniques have impacted the delivery of radiation for bladder cancer; discuss the role of ART, and potential dosimetric and clinical advantages of this emerging treatment strategy; and explore future directions for adaptive radiation for bladder cancer.
DISCUSSION
Impact of Evolving Technology on Radiation Delivery for Bladder Cancer
Prior to the development of CT scans, radiation treatment plans were initially designed based on two-dimensional imaging or plain X-ray films. The invention of CT imaging revolutionised radiation treatment delivery by providing three-dimensional visualisation of treatment isodose lines, termed 3D conformal radiation therapy. Furthermore, the integration of advanced imaging capability with medical linear accelerators brought forth two major technological advancements in radiation treatment. Firstly, image-guided radiation therapy emerged, and provided the treatment team with the ability to frequently reimage the patient with CBCT scans during the course of radiation treatment, to verify the position of the target, improving accuracy and precision of treatment delivery. Secondly, intensity-modulated radiation therapy (IMRT) was developed as an advanced form of 3D conformal radiation therapy, in which the intensity of each beamlet within a given treatment beam can be modulated with its own individual intensity level, leading to the construction of a complex pattern of dose delivery. This technique yields a highly conformal dose distribution, with improved precision of dose to target, and reduction of dose delivered to normal tissue.10
The widespread availability of CBCT imaging enabled daily visualisation of the bladder target throughout treatment delivery. This ability to visualise the bladder target every day prior to treatment made it clear to treating physicians that the bladder size and shape could change drastically between sessions, such that the ability of the original treatment plan, based on the initial planning CT scan, to adequately cover the target and spare surrounding normal tissues, could be compromised. Such observations led to the development of ART techniques. ART provides the opportunity to individualise and optimise treatment through the utilisation of images acquired either during, or immediately prior to, the treatment delivery, to modify the treatment plan based on specific anatomical patient factors. The rationale to support adaptive strategies is to more closely match the treatment volume created by the physician to the target volume revealed in today’s image. This results in an improvement in accuracy of treatment dose delivery to the target, and minimisation of dose to normal tissue. In the remainder of this review, the authors will discuss different techniques available for bladder cancer ART, discuss dosimetric and clinical advantages to ART, and explore future directions.
Important Radiation Planning Definitions
Before there can be more detailed discussion, it is important to define key terms specific to radiation treatment planning that will be utilised throughout this article. There are three types of target volumes that are used for radiation planning: gross tumour volume (GTV), clinical target volume (CTV), and planning target volume (PTV). The GTV describes the volume that can be seen and measured on a scan; the CTV describes the suspected microscopic extension of disease based on biologic data specific to disease site; and the PTV is a margin of volume added to account for uncertainty in targeting, for example, due to organ movement. In general, radiation oncologists begin by creating a GTV to define the gross visible disease. A margin is then added to the GTV to create a CTV that accounts for microscopic extension, and an additional margin is added to create a PTV that accounts for motion.
A second key concept in radiation treatment planning is minimisation of dose to healthy tissue near the target volume. Based on the target of interest, there are specific healthy tissues termed ‘organs-at-risk’ (OAR) that are near the target volume, and are likely to receive some radiation dose. When discussing radiation to the bladder, the rectum and the small bowel are the two key important OAR, and as a result, dose to those areas is carefully measured and monitored during radiation treatment. One key metric for the strength of a specific radiation technique is a reduction in healthy tissue volume that receives a high dose of radiation. When referring to volume receiving a certain dose of radiation, an acronym such as V50 Gy may be used, which means volume receiving a dose of at least 50 Gy. Understanding these definitions is important, as adaptive techniques in bladder cancer are commonly evaluated based on their ability to decrease the size of PTV margins, leading to reduction in V50 Gy delivered to the rectum, and V45 Gy delivered to the small bowel.
Adaptive Radiotherapy: Available Techniques
There are many different adaptive techniques reported in the literature, which can be split up into offline strategies, such as composite ART, and online strategies, including PoD and MR- or CBCT-guided daily plan re-optimisation (Figure 1).
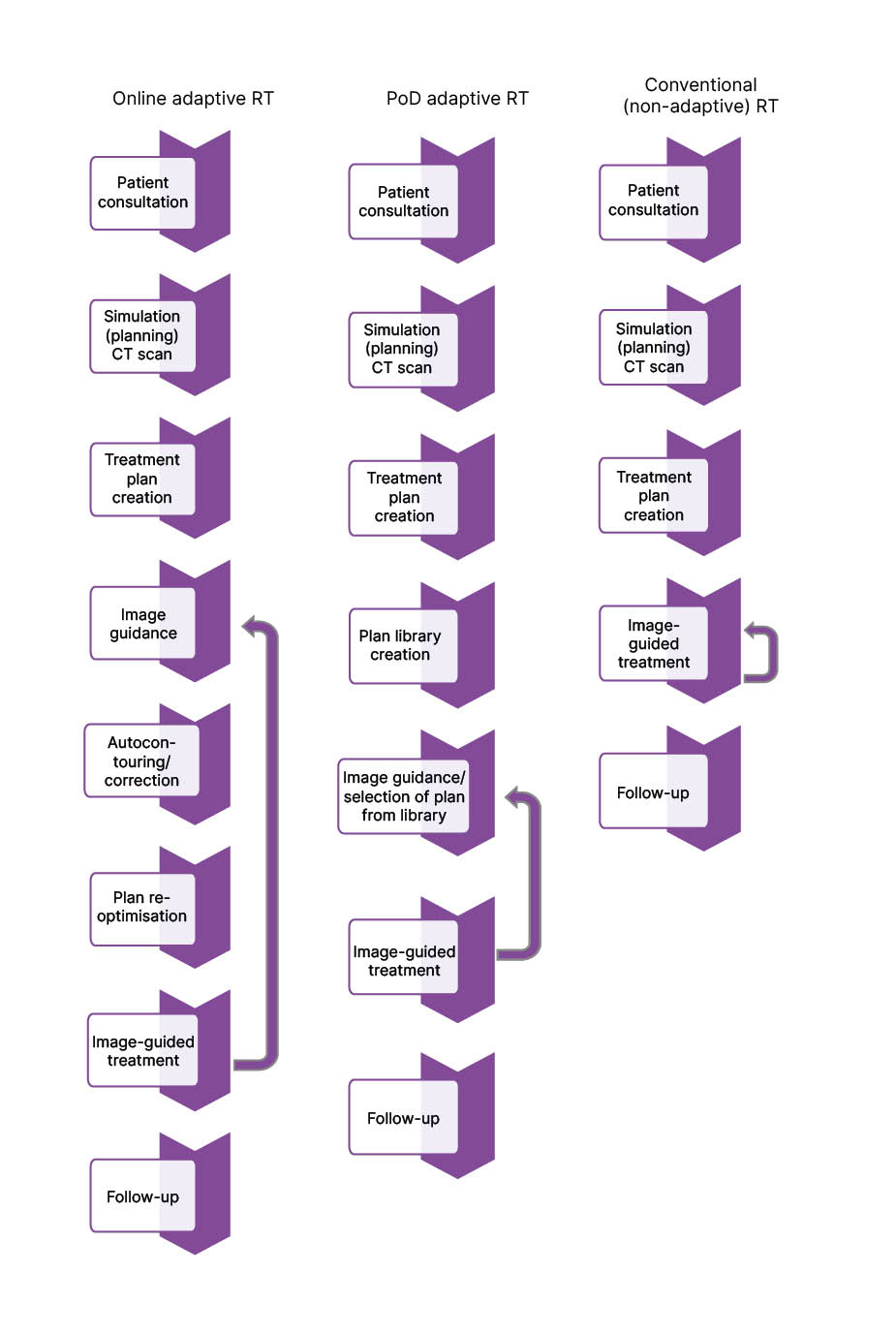
Figure 1: Workflow diagrams for conventional (non-adaptive) radiotherapy, plan-of-the-day adaptive radiotherapy, and online adaptive radiotherapy.
The arrows indicate processes that are repeated every session throughout the treatment course.
PoD: plan-of-the-day; RT: radiotherapy.
An offline composite ART plan involves using CBCT imaging from the first week of treatment to create one new adaptive plan based on the changes in patient’s anatomy seen during the week with PTV margin derived from the scans. Compared with conventional techniques, composite ART plans allow for the creation of a planning target volume that is more representative of the patient’s bladder size, shape, and position during treatment, leading to reduced PTV volume, higher conformity index (that is, the degree to which the treatment plan conforms to the target), and reduced toxicity, with similar target volume coverage.11,12 However, one primary limitation of offline ART is it can only provide a reduction in systematic error, and cannot account for unpredictable daily changes in bladder volume.
The PoD ART technique is one of the earliest online adaptive strategies, and therefore, the most studied and represented approach in the literature. This technique is conducted in two stages. Firstly, during the treatment planning stage, a library of three to five different plans is created, based on probabilistic, population-based margins for intra-fraction variation in bladder volume.13 Then, once treatment begins, CBCT images are acquired daily with the patient on the treatment table to assess patient anatomy, and a plan is selected in real time from the library that best represents the patient’s current anatomy.14 One specific variation to this approach further individualises the treatment by utilising CBCT images from the first week of treatment to generate a personalised library of plans, with margins based on the patient’s own bladder volume variability, rather than population-based margins.15 While this technique confers many of the dosimetric and clinical advantages that will be discussed in detail in this article, it is important to consider several limitations, including observer variability in plan selection, the potential that there is no suitable plan, increased treatment time, and logistical implementation error leading to geographic misses.12
More recently, the introduction of online re-optimisation using MRI-guided (MRgRT) or CBCT-guided systems has addressed some of the limitations of the PoD approach. MRI can improve the soft tissue contrast to refine target and OAR delineation.16 In addition, MRgRT can observe and account for intra-fractional bladder variability through real-time motion mitigation. The bladder is relatively mobile, and increases in size non-uniformly with filling. The magnitude of this change is rarely consistent, and current drinking protocols, catheterisation, dietary modifications, and laxatives have not shown consistent results in reducing bladder size variation.17,18 Thus, stochastic variation in organ filling, deformation, and peristaltic motions of the target and OAR can be monitored prior to MRgRT session, and the treatment plan adjusted accordingly.19 However, this approach is not without its own limitations, one of which is longer treatment times in the region of 30–40 minutes or longer.20
There have been two MR-guided linear accelerators introduced to the market, and in clinical use over the last decade, the ViewRay MRIdian® (GenesisCare, Sydney, Australia) and the Elekta Unity (Elekta, Stockholm, Sweden). Although they differ in some ways, they both use MRI for patient setup, and to track changes in the target in real time during the procedure. The ViewRay company recently declared bankruptcy and subsequently ceased operations, so this device is no longer in clinical operation. In part as an effort to minimise the time and resources necessary to conduct daily plan re-optimisation, the Ethos™ CBCT-guided ART system (Varian, a Siemens Healthineers Company, Palo Alto, California, USA) was developed more recently to take advantage of artificial intelligence (AI) and machine learning to optimise the ART workflow.21 This system has three key components: an automated treatment planning system for plan generation, online ART planning system with integrated quality assurance, and offline monitoring treatment solution.22,23 During treatment planning, the physician defines clinical goals that take into account fractionation (that is, how many sessions, or fractions, are used to deliver the total dose), and dose constraints on targets and OAR. The system applies the prioritised list of clinical goals and automatically generates a treatment plan. Once a treatment plan has been generated, online ART workflow uses iterative CBCT, or enhanced quality CBCT images, as input into an AI-driven program, which can re-calculate and re-optimise a new plan based on updated anatomy of that day. Plans can then be evaluated by the physician to select the best treatment plan.22 Although in a broad sense the on table ART workflow for the MR-guided and CT-guided systems are the same, treatment sessions are generally shorter using a CT-guided system. Studies have shown sessions conducted within a 15–25-minute time slot, although many variables may affect workflow timing, and determine the duration of treatment.23,24
In the setting of these various techniques, ART has several potential advantages over conventional, nonadaptive radiation, including improved dosimetric metrics, such as better target coverage and decreased dose delivery to healthy tissue, and clinical outcome measures, including disease control and toxicity mitigation. From the perspective of the patient, ART and non-ART procedures differ minimally. Patient instructions would include explaining the reason for the waiting time during the adaptation phase of the daily session (indicated in Figure 1 by the ‘Autocontouring/correction’ and ‘Plan re-optimisation’ stages of the treatment process) and re-emphasising the need to remain still as possible throughout. In the remainder of this review, the authors will discuss dosimetric benefits and clinical outcomes reported in the literature. A summary of findings from included studies can be found in Table 1.
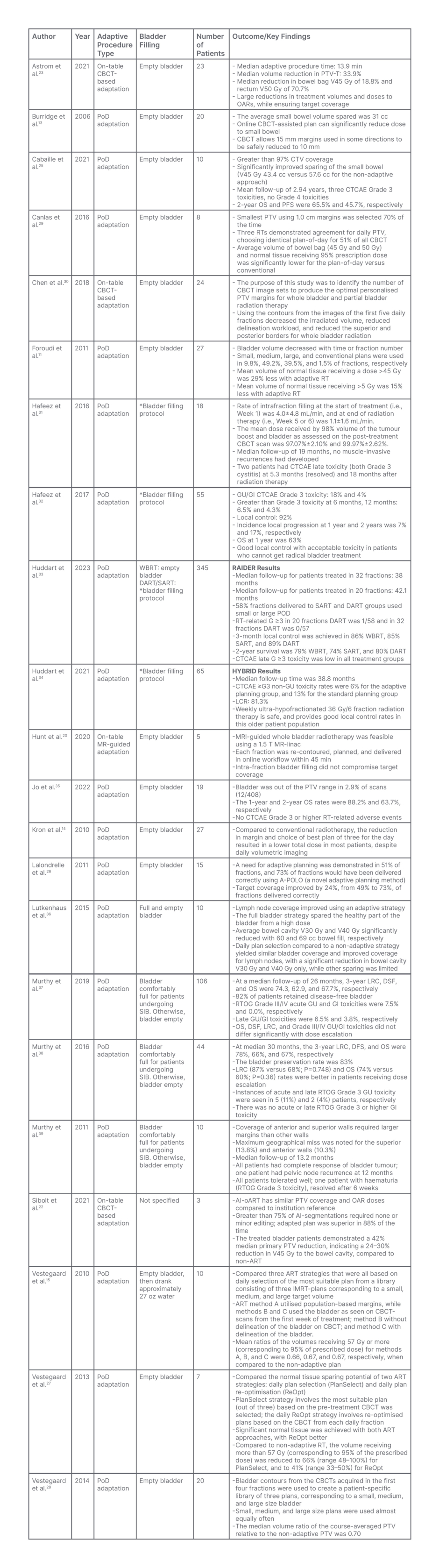
Table 1: Trials of daily adaptive radiotherapy for bladder cancer, including the procedure used for adaptation, state of bladder filling at treatment session start, number of patients involved, and clinical outcomes and key findings.
AI-oART: artificial intelligence – online adaptive radiotherapy; ART: adaptive radiotherapy; CBCT: cone-beam CT; CTCAE: Common Terminology Criteria for Adverse Events; CTV: clinical target volume; DART: dose-escalated adaptive tumour boost radiotherapy; DFS: disease-free survival; GI: gastrointestinal; GU: genitourinary; IMRT: intensity-modulated radiation therapy; LRC: locoregional control; MR: magnetic resonance; OAR: organs at risk; OS: overall survival; PFS: progression-free survival; PoD: plan-of-the-day; PTV: planning target volume; PTV-T: primary PTV; RT: radiotherapy; RTOG: Radiation Therapy Oncology Group; SART: standard dose adaptive tumour-focused RT; SIB: simultaneous integrated boost; V## Gy: volume of of tissue that is getting ## Gy of radiation dose (i.e., ‘bowel cavity V45 Gy was 60 cc’ means the volume of bowel cavity receiving 45 Gy of radiation was 60 cc); WBRT: standard whole bladder RT.
*After voiding, the patients drank 350 mL of water, 30 minutes after which a non-contrast-enhanced planning CT scan was performed (CT30), followed 30 minutes later by a second planning CT scan (CT60). No bladder emptying or drinking was permitted between the two scans.
Adaptive Radiotherapy: Dosimetric Benefit
There are many studies that report dosimetric benefits through improved PTV and CTV coverage using the PoD approach.13,15,25-28 Specifically, a British study looking at 20 patients with bladder cancer who underwent PoD ART found that online CBCT-assisted plan selection techniques allowed for 15 mm margins to be safely reduced to 10 mm.13 Another study in France looking at data from 10 patients treated for localised muscle invasive bladder cancer with PoD ART demonstrated a greater than 97% CTV coverage.25 Furthermore, a study examining patterns of bladder wall motion and validating a specific novel adaptive planning method called adaptive-predictive organ localisation (A-POLO) found that this strategy improved target coverage by up to 24%, increasing the percent of fractions delivered correctly from 49% to 73%.26 A-POLO is a unique version of PoD ART in which CT planning scans are taken at 0, 15, and 30 minutes after voiding, to create a ‘library’ of plans based on patient-specific bladder filling patterns. Finally, three studies conducted by Vestergaard and colleagues15,27,28 together support an improvement in PTV coverage, with no target compromise, improved target conformity, and reduction in PTV by 183 cm3 with PoD approach compared with non-adaptive techniques.
Another important advantage of ART is the sparing of normal tissue dose, and subsequent reduction in treatment toxicity. Many studies report improved sparing of bowel dose volume with ART.11,14,25,29,30,35,36 Canlas et al.29 compared dosimetric results between PoD ART and conventional treatment, and found that average volume of bowel bag and normal tissue receiving 95% of prescription dose was significantly lower for PoD versus conventional (p<0.01). Additionally, Cabaillé et al.25 reported a 43.7% average volume of healthy tissue spared with a 14.2 cc absolute reduction in small bowel V45 Gy, and 1.7 cc absolute reduction rectal V50 Gy for the adaptive versus the standard approach. Finally, Foroudi et al.11 demonstrated that more normal tissue was irradiated using conventional CTV planning compared with ART. More specifically, they showed that ART had 29% less mean volume of normal tissue receiving greater than 45 Gy. Together, these data provide compelling evidence that bladder ART strategies lead to a better conformity index, that yields improved target coverage and less dose to OAR, compared with conventional planning.
While data on CBCT-guided, AI-driven ART are more limited than PoD, there is evidence to support even better dosimetric outcomes with this newer technology. A study looking at pre-treatment planning for 39 pelvic cases, 100 online ART simulations, and five clinical online ART patients conducted on Ethos™ found that auto-generated treatment plans had similar PTV and OAR doses, with more than 75% AI segmentations requiring no or minor editing, and adaptive plan superiority in 88% of cases.22 Furthermore, the workflow for the first five treated patients had a total procedure time of 17.6 min, with 42% median primary PTV reduction, and 24–30% reduction in V45 Gy to the bowel cavity, compared with non-ART.22 Additionally, a study which analysed 297 fractions delivered with online ART full re-optimisation of anatomy showed a median online ART procedure time of 13.9 min, 33.9% median PTV volume reduction, 18.8% median reduction of V45 Gy to the bowel bag, and 70.7% median reduction of V50 Gy to the rectum.23 Overall, the data suggest that CBCT-guided online ART is feasible for patients with bladder cancer, AI-driven solutions are time-efficient, and this technique translates to PTV reduction and reduced dose to OAR.
Adaptive Radiotherapy: Clinical Outcomes
As discussed above, significant dosimetric improvements are achieved with the use of ART in urinary bladder irradiation. However, what is more important is whether these advances translate into improved clinical outcomes. Clinical outcomes can be split into two categories: disease control and toxicities. From a disease control perspective, there is evidence to support good local control, overall survival (OS), and progression-free survival (PFS) with online ART techniques. A French clinical study of 10 patients with muscle invasive bladder cancer undergoing PoD ART with a median follow-up of 2.94 years showed 2-year OS and PFS were 65.5% and 45.7%, respectively. Two out of the 10 patients had locoregional relapse, while another three patients developed metastatic diseases.25 Hafeez et al.31 reported a group of 18 patients with muscle-invasive bladder cancer of any histologic subtype treated with whole bladder to 52 Gy and simultaneous integrated boost to the tumour to 70 Gy in 32 fractions. In this study, median follow up was 19.0 months, with no evidence of recurrence.31 The same group in London, UK, also reported on 55 patients with T2-T4aNx-2M0-1 bladder cancer who were treated with 36 Gy in 6-weekly fractions and demonstrated 92% local control with OS of 63% at 1 year.32 Furthermore, several single arm studies conducted between 2011–2022 have reported similar survival rates.35,37-39 In addition, Jo et al.35 described eight of 19 patients developed local recurrence at 1 year post-treatment. Murthy et al. reported a 3-year locoregional control rate of 78%.38
Prior to 2021, there were no randomised control data comparing standard, non-adaptive strategies with ART for bladder cancer. In the last decade, two protocols were developed to address this gap in the literature. The HYBRID protocol compared a single-standard plan (non-adaptive) to adaptive planning in patients with T2-T4aN0M0 muscle invasive bladder cancer receiving 36 Gy in 6-weekly fractions. This study enrolled 65 patients with a median follow-up time of 38.8 months, and found a local control rate of 81.3%.34 Furthermore, at the 2023 American Society of Clinical Oncology (ASCO) meeting, preliminary data from the RAIDER protocol, which compared standard whole bladder radiotherapy to standard dose adaptive tumour-focused radiotherapy and dose-escalated adaptive tumour-focused radiotherapy in 325 patients with T2-T4aN0M0 unifocal muscle invasive bladder cancer, were presented. This abstract reported local control rates of whole bladder, standard dose adaptive tumour-focused, and dose-escalated adaptive tumour focused radiotherapy were 86%, 85%, and 89%, respectively, and 2-year survival rates were also similar among the three treatment groups.33
The dosimetric benefits of sparing normal tissue translate into low rates of significant Grade 3 and 4 toxicity. The toxicity criteria of the Radiation Therapy Oncology Group (RTOG) and the European Organization for Research and Treatment of Cancer (EORTC) were described in the referenced editorial.40 In general, side effects from radiation are considered in the context of acute and late toxicity in the specific location of treatment. Given the location of the bladder in the pelvis, the most common side effects are gastrointestinal (GI) and genitourinary. In the acute setting, common side effects include fatigue, increased frequency or urgency of urination, and change in frequency or quality of bowel habits, including diarrhoea. In the longer term, toxicity could include cystitis, GI bleeding, or GI/genitourinary obstruction, including urinary stricture.41,42 Delving into toxicity data, Cabaillé et al.25 reported Grade 3 toxicities in three patients, but no Grade 4 toxicities, and Hafeez et al.31 reported Grade 3 toxicity in two patients at 5.3 months and at 18.0 months after receiving radiation therapy.31 The other single arm studies report Grade 3 toxicity rates up to 18%, with no Grade 4 toxicity.32,35,37-39 When looking at data from the two randomised control trials, RAIDER reported only one case of Grade 3 radiotherapy-related toxicity in patients in the adaptive arm,33 and HYBRID reported Grade 3+ toxicity rates of 6% with adaptive techniques compared with 13% in standard radiation.34
FUTURE DIRECTIONS
This review summarises data that have shown improvements in radiotherapy by reducing dose to normal tissue, improving target coverage, and potentially enhancing clinical outcomes. However, most of the current existing data are based on retrospective or small sample size single arm cohort studies, with limited larger scale prospective data on clinical outcomes. Currently, there are nine ongoing clinical trials around the world investigating clinical outcomes of bladder ART, including two prospective trials comparing intestinal toxicity ART and non-adaptive IMRT (NCT05295992, NCT05700227);43,44 one trial in Canada looking at feasibility, treatment plan quality, and toxicity of ART approach for bladder and prostate cancer (NCT03909893);45 one trial in the UK determining maximum tolerated dose of external beam radiation therapy delivered as tumour boost in patients who have undergone transurethral bladder resection for muscle invasive bladder cancer (NCT01124682);46 and three additional trials studying feasibility, local control, and toxicity of various ART techniques (NCT01810757, NCT01000129, NCT05833867).47-49 Furthermore, there are two ongoing clinical trials investigating clinical outcomes and patient-reported outcomes with the use of EthosTM CBCT-based ART in bladder cancer (NCT05295992, NCT05700227).43,44 These studies are designed to demonstrate meaningful improvements in treatment-related side effects by comparing ART techniques with standard of care non-adaptive IMRT plans.
CONCLUSION
The development of ART treatment planning techniques for bladder radiation therapy has emerged as a promising solution to improve treatment delivery precision in the setting of daily changes in a patient’s bladder anatomy throughout the treatment course. There are many ART techniques reported in the literature, each with their own unique advantages and disadvantages. With the ability to adjust and modify treatment plans prior to delivery, ART provides dosimetric gains, such as improved target coverage and decreased dose to normal tissue, and clinical benefits, such as improved local control and decreased toxicity. Ongoing prospective trials will provide stronger, more robust clinical data on ART for bladder cancer, to better define clinical outcomes in terms of disease control, toxicity, and patient-reported outcomes.