Abstract
Whole-body magnetic resonance imaging (WB-MRI) has significantly improved oncological disease management by enabling comprehensive visualisation of the entire body in a single scan, facilitating the diagnosis, staging, and monitoring of various cancers. Its integration with diffusion-weighted imaging and apparent diffusion coefficient mapping offers quantitative insights into tumour biology, notably enhancing treatment planning and response evaluation. The evolution from 2D to 3D WB-MRI has improved image resolution and reduced scan times. This review underscores the pivotal role of WB-MRI in oncology, highlighting its efficiency in detecting and characterising tumours and assessing treatment response with a focus on 3D techniques.
Key Points
1. In oncology, whole-body magnetic resonance imaging (WB-MRI) plays an invaluable role in tumour detection, characterisation, and assessment of treatment response, offering a fast, comprehensive assessment of multiple body parts.
2. 3D WB-MRI techniques have improved WB-MRI with respect to image resolution, allowing multiplanar visualisation and improved detection with faster scan times.
3. With the addition of diffusion-weighted imaging, WB-MRI is an alternative to PET-CT, avoiding radiation exposure, and furthers the ability of MRI to characterise detected lesions.
INTRODUCTION
As a safe and comprehensive imaging modality that visualises a wide area of the body, whole-body magnetic resonance imaging (WB-MRI) plays an invaluable role in diagnosis, staging, surveillance, and assessment of response to treatment in various diseases, most notably in oncology.1 With WB-MRI, one imaging study can be performed instead of multiple local MRI examinations of different body parts. Employment of WB-MRI in the management of patients with cancer has been associated with lower healthcare costs and shorter staging times.2
WB-MRI has been widely employed in the staging of bone and soft tissue malignancies.3-7 WB-MRI also aids in the assessment of tumour burden needed for treatment planning in multiple myeloma, lymphoma, and neurofibromatosis.8-10 In cases of metastatic cancers, WB-MRI can visualise tumour deposits throughout the body with superior efficacy compared to conventional imaging modalities, for both bone and soft tissue disease, and the role of WB-MRI has been studied in the management of various metastatic cancers including prostate, lung, breast, and ovary.3,11-14
The addition of functional imaging with diffusion-weighted imaging (DWI) and apparent diffusion coefficient (ADC) mapping to WB-MRI protocols provides quantitative insight into the management of oncologic patients.15 DWI sequences assess the Brownian movement of water molecules in the tissues and have been used to differentiate between malignant and benign lesions throughout the body.16 Treatment planning, the assessment of response to neoadjuvant treatment, and surveillance for disease recurrence are other advantages of WB-MRI with DWI.17,18
WB-MRI sequences are traditionally 2D sequences consisting of T1-weighted and fluid-sensitive sequences (with either T2-weighted images or short-tau inversion recovery (STIR) sequences), although for some protocols the WB-STIR is used alone.19 2D WB-MRI is performed with image acquisitions in different planes, although often the coronal plane is prioritised to reduce overall acquisition time; with 2D WB-MRI, information is limited to the plane of acquisition. However, with 3D WB-MRI, images are obtained in a single acquisition (typically in the coronal plane) with isotropic or almost-isotropic resolution, and images are reformatted to other planes of interest such as the axial or sagittal planes.9 3D acquisition therefore renders the duration of WB-MRI significantly shorter and offers higher spatial resolution.19 Furthermore, 3D WB-MRI allows for a volumetric measurement of the tumours providing more accurate sizing and quantitative assessment of the tumour burden throughout the body.20 Despite the advantages, 3D WB-MRI also has disadvantages, the most salient being that it is more vulnerable to motion artefacts. Because the images are reconstructed, patient movement during the scan impacts all the subsequent reconstructed images.
In this review article, the applications of WB-MRI in musculoskeletal oncology are discussed, with a focus on the development of 3D WB-MRI and the role of WB-MRI in the management of musculoskeletal oncologic diseases.
EVOLUTION OF WHOLE-BODY MRI IN ONCOLOGY
The application of WB-MRI in oncologic settings is rising.21 A whole-body approach incorporated with the high-quality MRI of bone and soft tissues makes WB-MRI an excellent modality for the management of various types of cancers. For example, WB-MRI has an established role in the management of cancers with bone metastases.17,22-25
2D WB-MRI has been the conventional method of WB-MRI and usually includes T1-weighted and STIR sequences in the coronal plane.26 Due to single-planarity, 2D WB-MRI fails to provide high-resolution images in other planes. Adding multiple planes, on the other hand, increases the scan time significantly and is typically not done for clinical examinations.27
The advent of 3D MRI and functional MRI techniques have made WB-MRI a reliable alternative for previously established whole-body diagnostic methods, in particular CT and PET scans.3 By acquiring the images in a single plane and reconstructing them into multiplanar 3D images, 3D MRI offers shorter acquisition time which may translate into increased patient satisfaction and a decreased chance of motion artifacts, all while avoiding radiation exposure imparted by PET and CT imaging.27
CURRENT 3D WHOLE-BODY MRI SEQUENCES
3D T1-Weighted Fast Spin Echo
3D T1-weighted fast spin echo (FSE) sequences have been developed by a variety of vendors and most widely include 3D turbo spin-echo sampling perfection with application-optimised contrast using different flip angle evolutions (SPACE, Siemens Medical Systems, Erlangen, Germany) as well as CUBE (General Electric, Boston, Massachusetts, USA) and volume isotropic turbo spin echo acquisition (VISTA, Philips, Amsterdam, the Netherlands). The SPACE method has been particularly studied for WB-MRI; this sequence has been shown to enhance the signal-to-noise ratio (SNR) significantly compared to conventional 2D T1-weighted WB images.26 To further shorten the acquisition time and increase the isotropic resolution, Controlled Aliasing in Parallel Imaging Results in Higher Acceleration (CAIPIRINHA), a parallel imaging technique, has been employed for 3D imaging.28
3D Gradient Echo
In addition to 3D FSE sequences, a variety of vendors have also developed ultrafast 3D T1-weighted gradient echo sequences (Figure 1), mainly for post-contrast imaging (Figure 2 and 3). These were originally used in abdominal imaging and provide high-spatial resolution images during breath holding for a short period of time.29 These types of sequences are based on T1-weighted, fat-suppressed images and have short repetition time (TR). The short TR, utilisation of uneven k-space sampling, and zero-filling interpolation in the section-select direction allow for high-resolution, isotropic images with an overall shortened scan time,29 all ideal for contrast-enhanced imaging. This 3D WB MRI technique has been proposed as a reliable method for tumour staging,4,30 with comparable diagnostic capabilities to conventional 18F-fluorodeoxyglucose (18F-FDG) PET/CT techniques in the evaluation of recurrence of non-small cell lung cancer.13 Furthermore, dynamic contrast-enhanced images can be obtained using 3D sequences; dynamic contrast-imaging has shown promising results in metastasis detection in various types of cancer.30
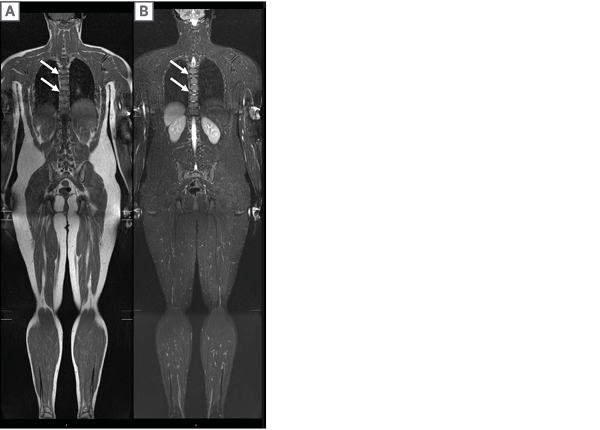
Figure 1: A 13-year-old male with T5 and T8 vertebra plana (arrows).
An efficient 2D WB-MRI, comprised of A) coronal STIR and B) T1 weighted images, was performed to evaluate other sites of diseases. Due to single-planarity, 2D WB-MRI fails to provide high-resolution images in other planes and each additional plane of image requires a separate acquisition and additional scan time.
STIR: short tau inversion recovery; WB-MRI: whole-body magnetic resonance imaging.
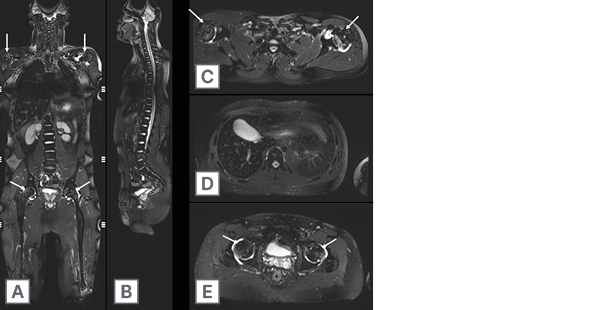
Figure 2: A 24-year-old male with multifocal osteonecrosis.
Single coronal 3D isotropic volumetric SPACE (Siemens Medical Systems, Erlangen, Germany) STIR sequence A) enables the generation of high-resolution multiplanar reformations in the B) sagittal and C–E) axial plane. Note osteonecrosis (arrows) involving the bilateral mid to distal femoral diaphysis extending into the epiphyses, bilateral proximal tibia extending into the epiphyses, bilateral humeral heads with reactive bilateral glenohumeral and hip joint effusions.
STIR: short tau inversion recovery.
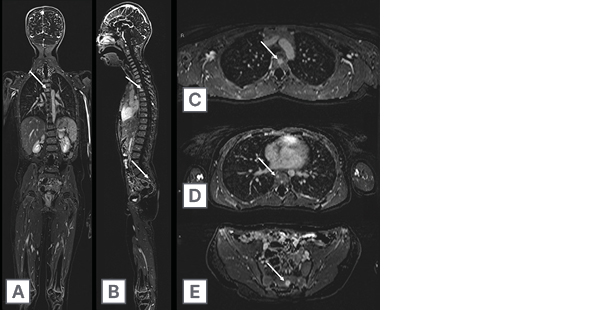
Figure 3: An 8-year-old child with history of germline RB1 mutation and at high risk for sarcomas and soft tissue tumours.
Annual surveillance whole body MRI comprised of 3D sequences including post-contrast 3D T1 GRE-based FS subtraction images acquired in the A) coronal plane with multiplanar reformations in the B) sagittal and C–E) axial plane. Note several skeletal lesions (arrows) within the thoracic spine and sacrum.
FS: fat-suppressed; GRE: gradient echo.
3D T1-Modified Dixon Gradient Echo
A 3D modified Dixon (mDixon) sequence has been proposed as an addition to the WB-MRI protocol to further shorten the acquisition time. Essentially an anatomical T1-weighted sequence, 3D mDixon gradient echo (GRE) utilises a modified 2-point Dixon fast field echo platform to reconstruct in-phase, out-of-phase, water, and fat images.31 This method significantly decreases the acquisition time while maintaining an acceptable diagnostic performance. Also, the reconstructed fat images of 3D WB mDixon GRE have been shown to have a superior tissue-lesion contrast compared to the 3D WB FSE method.31 Relative fat-fraction mapping is a technique that relies on both ultrafast GRE and Dixon sequences to produce images using the water and fat images of Dixon. This technique has shown promising results in detecting bone marrow lesions in various metastatic cancers.32
3D T2-Weighted/ Short-Tau Inversion Recovery
STIR sequences have inherent capabilities to produce both T1- and T2-weighted images.9 Most notably, STIR often offers superior and more uniform fat signal nullification compared to fat-suppressed T2-weighted sequences.19 2D STIR has been extensively included in WB-MRI scans of various cancers.9,33,34 The combination of T2-weighted FSE SPACE sequence with STIR allows for high-resolution, 3D fat-saturated images that can be reconstructed in multiple planes to improve the identification of lesions of bone marrow or other fat-surrounded lesions.35 Employment of 3D T2-weighted STIR in WB-MRI has shown promising results in the detection of bone marrow metastasis.36 Notably, some authors have reported an equal diagnostic performance of a single 3D T2-weighted WB STIR sequence compared to a WB MRI protocol of T1-weighted, DWI, and 3D T2-weighted WB STIR in paediatric patients (Figure 4), thus reducing the acquisition time for WB MRI in children.37
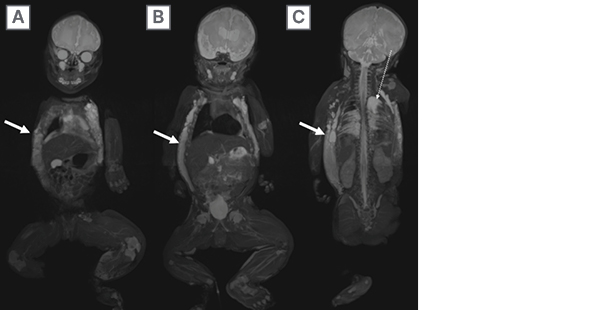
Figure 4: A 5-day-old infant with a clinically visible vascular malformation in the chest and left lower extremity hemihypertrophy.
A–C) Sequential 3D SPACE (Siemens Medical Systems, Erlangen, Germany) STIR WB-MRI reveal extensive right greater than left chest wall and flank lymphatic malformation (solid arrows) with left chylothorax (dashed arrow). Given associated asymmetric left lower extremity hypertrophy, the patient was diagnosed with Klippel-Trenaunay syndrome.
STIR: short tau inversion recovery; WB-MRI: whole-body magnetic resonance imaging.
3D Diffusion-Weighted Imaging
DWI with ADC mapping is a functional imaging technique that offers useful information for detecting and characterising lesions, through the study of the diffusion of water molecules in the target tissues (Figure 5). As an isotropic sequence, DWI can be readily reformatted into multiple planes as well as provide 3D images.25 DWI with ADC mapping complements a WB-MRI protocol by aiding in lesion detection, characterisation, and assessment of response to treatment in various oncologic diseases.17,24,38,39 DWI also shortens the WB-MRI interpretation time.40 Moreover, by precisely predicting the resectable lesions, WB DWI has been shown to be decisive in the treatment planning of patients with cancer.41
The combination of single-shot spin-echo echo-planar DWI with STIR yields a sequence called DWI with background body signal suppression (DWIBS).42 DWIBS is a technique that allows the suppression of signal from the tissues in the background of the target lesion and is useful for detection, staging, and follow-up of neoplastic disease.43,44 It has also been shown to be approximately 80% less expensive than the alternative whole-body test, FDG-PET/CT, for the detection of metastatic disease.45
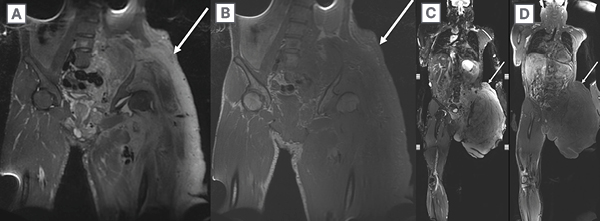
Figure 5: A 27-year-old man with neurofibromatosis Type 1 and extensive multi-spatial superficial and
deep left lower extremity plexiform neurofibroma.
Neurofibromatosis Type 1 and extensive multi-spatial superficial and deep left lower extremity plexiform neurofibroma (arrow) that is incompletely imaged on A) coronal 2D STIR and B) T1 weighted images as it traverses traditional imaging planes. Subsequently, the patient underwent WB-MRI using C) coronal SPACE (Siemens Medical Systems, Erlangen, Germany) STIR and D) T1-weighted images. WB-MRI enables the determination of overall tumour burden in a single imaging session.
STIR: short tau inversion recovery; WB-MRI: whole-body magnetic resonance imaging.
FIELD STRENGTH CONSIDERATIONS: 1.5T VERSUS 3.0T MRI
The choice between 1.5T and 3.0T MRI systems has implications for image quality, scan time, and susceptibility to artefacts. While 3.0T MRI systems offer higher SNR and potentially higher spatial resolution, they are also more susceptible to artefacts, particularly with DWI sequences.4 In practice, many centres find that DWI performs better and more consistently with fewer artefacts at 1.5T, especially in whole-body imaging where geometric distortions and susceptibility artefacts can be more pronounced at higher field strengths.46 Additionally, 1.5T systems may provide more uniform fat suppression and fewer artefacts from implants or metallic hardware. Therefore, the selection of field strength should consider the specific clinical application, patient population, and available equipment, balancing the desire for higher SNR against the potential for increased artefacts.
ROLE OF INTRAVENOUS CONTRAST WITH WHOLE-BODY MRI
While contrast-enhanced imaging can enhance the characterisation (as well as detection) of lesions, particularly with the use of T1-weighted fat-suppressed sequences, concerns regarding the accumulation of gadolinium-based contrast agents, especially in patients requiring frequent imaging, have led to the consideration of non-contrast protocols or gadolinium-sparing approaches.5,47 Non-contrast WB-MRI protocols, particularly those utilising DWI and STIR sequences, have been shown to be effective in the detection of metastatic disease and in the treatment response assessment.24,48,49 Furthermore, T1-weighted fast spin echo sequences, in combination with DWI, are advocated for the investigation of patients with myeloma, without the use of intravenous contrast. In patients who undergo serial imaging, such as those with genetic cancer predisposition syndromes or those undergoing surveillance for metastatic disease, minimising the use of gadolinium contrast agents can reduce the potential risks associated with gadolinium deposition.
For patients who do receive contrast-enhanced imaging, dynamic sequences such as 3D time-resolved imaging with stochastic trajectories can provide valuable information regarding lesion vascularity and enhancement patterns.6,50 These dynamic sequences can aid in the characterisation of lesions and assessment of treatment response, although they are limited to a regional evaluation (rather than the whole body). Additionally, subtraction techniques, where pre-contrast images are subtracted from post-contrast images, can improve lesion conspicuity and aid in the detection of areas of subtle enhancement (Figure 3).32
STANDARDISATION AND QUALITY CONTROL IN WHOLE-BODY MRI
Ensuring consistency and reproducibility in WB-MRI across different imaging centres and scanners is a significant challenge. Variations in scanner hardware, software, and imaging protocols, as well as radiologists’ comfort with particular sequences, can lead to differences in image quality and quantitative measurements, such as ADC values in DWI. To address these challenges, standardisation efforts include the use of standardised imaging protocols, phantom studies for quality assurance, and daily quality control (QC) procedures.
Phantoms, which are standardised objects with known properties, can be used to calibrate scanners and assess performance across different systems. Regular phantom imaging allows for the monitoring of scanner performance, identification of drifts or deviations, and correction of discrepancies between scanners.51 This is particularly important in multicentre studies or when patients are imaged on different scanners over time.
Daily QC procedures, including the assessment of image uniformity, SNR, and artefact evaluation, help maintain consistent image quality. Artefact mitigation strategies, such as patient coaching to minimise motion, the use of motion correction algorithms, and the application of advanced imaging techniques (e.g., parallel imaging, respiratory gating), can improve image quality and reduce artefacts.52
Finally, the development and adoption of standardised reporting systems, such as the METastasis Reporting and Data System for Prostate Cancer (MET-RADS), Myeloma Response Assessment and Diagnosis System (MY-RADS), and Oncologically Relevant Findings Reporting and Data System (ONCO-RADS) guidelines, also contribute to consistency in image acquisition, interpretation, and reporting.53-55 By adhering to standardised protocols and incorporating QC measures, the reliability and utility of WB-MRI in clinical practice can be enhanced, although serial imaging of patients is most efficient and effective when performed at the same centre with the same protocol in place. Table 1 summarises the currently used 3D WB-MRI sequences.
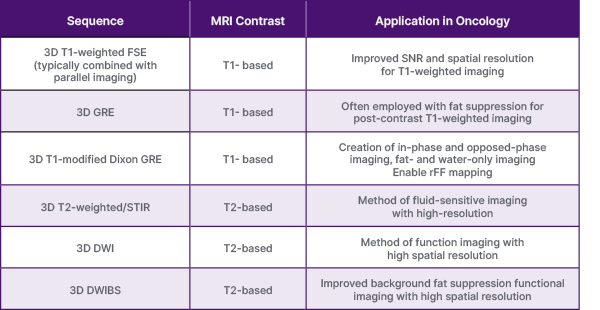
Table 1: Summary of 3D whole-body MRI techniques.
DWI: diffusion weighted imaging; DWIBS: diffusion weighted imaging with body background signal suppression;
FSE: fast spin echo; GRE: gradient echo; rFF: relative fat-fraction; STIR: short tau inversion recovery.
3D WHOLE-BODY MRI APPLICATIONS
Given its comprehensive nature and lack of need for radiation exposure, WB-MRI is an invaluable asset in the management of oncologic patients who are generally in need of multiple imaging studies over the course of their disease. Understandably, the applications of WB-MRI have been established in the treatment guidelines of some cancers, namely breast, prostate (MET-RADS), and multiple myeloma (MY-RADS).53,54,56 Also, WB-MRI can be an invaluable asset in the screening and surveillance of patients diagnosed with genetic cancer-predisposing syndromes such as NF-1, schwannomatosis, or Li-Fraumeni syndrome (LFS).33,57 Furthermore, the ONCO-RADS has been introduced to standardise the acquisition, interpretation, and reporting of WB-MRI in these oncologic diseases.55 The applications of 3D WB-MRI can be classified into the categories below.
Tumour Detection: Assessment of Tumour Burden and Metastatic Disease
WB-MRI has been widely utilised for the detection of bone and soft tissue tumours. Notably, genetic tumour predisposition syndromes can benefit from WB-MRI. These syndromes, such as NF-1, schwannomatosis, and LFS, commonly necessitate routine surveillance scans and tumour burden assessments (Figure 6). WB-MRI enables the determination of overall tumour burden and the detection of metastatic deposits in a single imaging session. The application of WB-MRI in NF-1 and schwannomatosis syndromes has shown promising results and has been recommended in the management of patients with NF-1 and higher tumour burden.9,38 Annual WB-MRI has also been recommended for cancer screening in patients with LFS.57
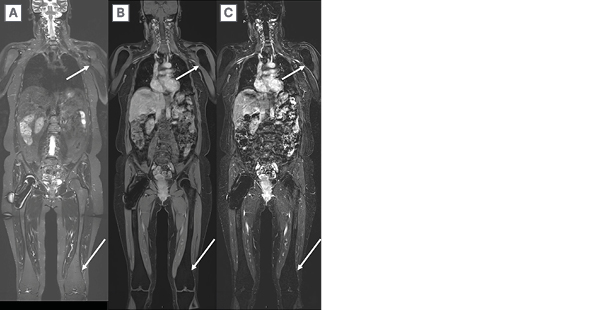
Figure 6: A 47-year-old adult with multiple hereditary exostosis.
A) Coronal 3D SPACE (Siemens Medical Systems, Erlangen, Germany) STIR and B) post-contrast T1-weighted, and C) post-contrast T1-weighted subtraction images reveal multifocal sessile osteochondromas (arrows) and distal femoral Erlenmeyer flask deformity but no suspicious features of malignant transformation.
STIR: short tau inversion recovery.
The diagnostic accuracy of 2D WB-MRI for the detection of metastatic disease in various types of cancers has been compared with PET/CT scans and studied to a greater extent than 3D WB-MRI, showing that the supplanting of 18F-FDG PET/CT with WB-MRI can also have positive economic implications.45 In their meta-analysis, Medeiros T et al.58 showed that a protocol of 2D WB-MRI with DWI has a comparable diagnostic efficacy in the assessment of metastasis in non-small cell lung cancer compared to 18F-FDG PET/CT.5 A study on 30 patients with naïve indolent lymphoma has shown comparable diagnostic accuracy between 2D WB-DWIBS/T2-STIR and 18F-FDG PET/CT in staging of the disease, although the authors have reported a higher sensitivity for WB-MRI for the detection of extra-nodal lesions.59 Similarly, a meta-analysis has found high sensitivity and specificity for 2D WB-MRI for the detection of nodal and extra-nodal lesions in patients with lymphoma.5
WB-MRI can aid in the detection of bone lesions, particularly with the use of a high b value DWI on which metastatic lesions will retain signals against normal background marrow elements. Notably, WB-MRI has superior sensitivity and specificity than other conventional techniques in visualising bone marrow malignant lesions.47 A comparable accuracy between 2D WB-MRI and 18F-FDG PET/CT for the detection of bone metastases in various types of cancer has been reported.48 For myeloma, a haematologic neoplasm involving bone marrow and causing osteolytic lesions, WB-MRI benefits are substantial in that it provides invaluable insight into the diagnosis of myeloma, even before the formation of osteolytic lesions.47
The assessment of bone metastasis in patients with prostate cancer is another utility of WB-MRI.24 Multiple studies have shown better diagnostic accuracy of WB-MRI compared to bone scintigraphy and equal efficacy as 18F-FDG PET/CT in the diagnosis of metastatic disease in patients with prostate cancer.60-62 However, studies on prostate-specific tracers such as 68Ga-Prostate Specific Membrane Antigen (PSMA) with PET/CT scans have shown preferable diagnostic performance compared to WB-MRI in the staging of metastatic prostate cancer.49
3D WB-MRI techniques have further improved WB-MRI with respect to image resolution, planes of acquisition, and scan time.19 A study on various cancer types that compared WB 3D VIBE MRI with scintigraphy showed outperformance of WB-MRI in diagnosing metastatic bone involvement.30 In patients with prostate cancer, 3D WB-MRI has shown comparable or better diagnostic performance with shorter acquisition time than 2D WB-MRI for the detection of bone and lymph node metastasis.19,63 Finally, for patients with breast cancer, WB-MRI protocols with 3D sequences have shown on par diagnostic outcomes compared with PET/CT scans in patients with a high risk of metastasis.6
Tumour Characterisation
The addition of DWI to a WB-MRI protocol enhances lesion detection by WB-MRI, particularly bone lesions (Figure 7). Yet, the importance of DWI is to improve lesion characterisation, once detected by WB-MRI.9,38 Using ADC maps, DWI can help quantitatively distinguish malignant from benign lesions, which is advantageous, especially in the management of genetic tumour predisposition syndromes where malignant degeneration amongst numerous benign tumours can occur.50 Serial imaging studies are crucial for early detection of malignant transformation in benign lesions, and WB-MRI with DWI provides a comprehensive overview of tumour deposits in the body. Debs P et. al.64 have reported comparable accuracy of WB-MRI compared to localised MRI in patients with NF-I and schwannomatosis, thus confirming that a protocol of WB-MRI with DWI sequence can replace multiple, local MRIs, which are necessary for surveillance of detected lesions. Although there is no standardised threshold, it has been widely accepted that malignant lesions tend to have lower ADC values.16
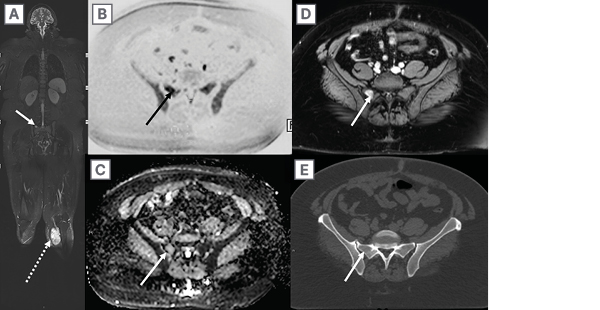
Figure 7: A 38-year-old woman with left lower extremity myxoid liposarcoma (dashed arrow on a)
underwent whole-body magnetic resonance imaging for staging purposes.
A) Coronal 3D SPACE (Siemens Medical Systems, Erlangen, Germany) STIR shows a right hemi-sacral skeletal lesion (arrow), which is conspicuous on B) inverted scale axial high b-value DWI, C) axial ADC map, and D) post-contrast 3D T1 GRE images through the pelvis. E) This lesion was occult on concurrent axial CT. As such, the patient underwent a biopsy, and this histologically confirmed as a site of myxoid liposarcoma metastasis. WB-MRI has superior sensitivity and specificity than other conventional techniques in visualising bone marrow malignant lesions, particularly with the use of a high b value DWI on which metastatic lesions will retain signal against normal background marrow elements.
ADC: apparent diffusion coefficient; DWI: diffusion weighted imaging; GRE: gradient echo; STIR: short tau inversion recovery; WB-MRI: whole-body magnetic resonance imaging.
Assessment of Treatment Response
WB-MRI can demonstrate changes in tumour size or composition. By employing DWI in particular, WB-MRI can aid in the assessment of treatment response for various bone and soft tissue malignancies. This application has been studied in myeloma, prostate cancer, and lymphoma.39,65-68 Besides a decrease in tumour size and signal intensity in T2/STIR images, which can signify a response to treatment, ADC mapping can provide a quantitative metric for determining treatment response in patients with myeloma.66,69 Post-treatment WB-MRIs have shown drastically higher ADC values after chemotherapy or autologous stem cell transplant, compared to pre-treatment scans.69,70 Increased ADC values are associated with intralesional necrosis, a feature of response to treatment,67 although tumours can also respond by forming post-treatment sclerosis rather than necrosis (also observed to have increased ADC values compared with viable tumours). Although the ADC values have not been standardised yet, post-treatment ADC values significantly correlate with standardised tracer uptake measures in PET/CT scans.67 DWI WB-MRI can accurately visualise response to radionuclide in metastatic bone lesions in patients with castration-resistant prostate cancer.39
FUTURE PERSPECTIVES IN ONCOLOGICAL IMAGING
Individualised management of oncologic patients is a novel approach and a trending topic in recent studies. A 3D WB-MRI scan is an invaluable modality for patient-tailored, thorough imaging of patients with cancer and has the potential to be further incorporated into the individualised medical management of breast, prostate, pancreatic, and colorectal cancers.71,23 In breast cancer, for example, WB-MRI has been shown useful to detect metastatic lesions with high sensitivity, aiding in staging and treatment planning.6,71 In prostate cancer, WB-MRI is well suited to evaluating both bone and soft tissue metastases, guiding decisions on systemic therapies.23,24 For colorectal cancers, WB-MRI can assess the extent of disease spread, which is crucial for surgical planning and determining eligibility for curative interventions.2
The integration of WB-MRI data into radiomics is another promising development in oncological imaging. Radiomics involves extracting a large number of quantitative features from medical images, such as texture, shape, and intensity, which can reveal patterns correlating with disease characteristics and patient outcomes.72 By applying advanced algorithms to WB-MRI data, radiomics can help predict tumour behaviour, likelihood of response to specific treatments, and potential for recurrence.73
Machine learning and AI, as well as almost every other aspect of radiologic studies, have impacted the WB-MRI acquisition and interpretation. As an emerging trend, the application of AI-driven methods such as deep learning or neural networks in WB-MRI studies has introduced faster WB-MRI scans while providing denoised images.74,75
LIMITATIONS AND CHALLENGES
Despite the benefits, 3D WB-MRI has some drawbacks. Like other 3D MRI techniques, 3D WB-MRI is vulnerable to patient motion during the scan. Also, bulk movements of the heart and the adjacent mediastinal components can result in artefacts, especially in soft tissue neoplasms such as lymphoma.18
Some authors have reported instances of false-positive or false-negative cases in paediatric neuroblastoma patients, thus lower accuracy of WB-MRI compared to PET/CT scan.76 However, recent studies have emphasised the diagnostic accuracy of WB-MRI and its comparability with PET/CT scans in paediatric patients.77
Inherent variations in MRI scanners and protocols can pose challenges when using WB-MRI to track complex patients over time, particularly when compared to PET/CT imaging, which is more standardised. To ensure consistency between acquisitions and across different scanners, additional centre-specific work is required to standardise the imaging protocols and calibration. This includes the use of phantoms for calibration, adherence to standardised imaging protocols, and regular QC procedures.51 Despite these efforts, some variability may remain, and radiologists must be aware of these limitations when interpreting serial WB-MRI studies.
Although variable in different parts of the world, the cost of WB-MRI should be considered as a limiting factor in its application.78 Ultimately, the interpretation of WB-MRI scans might be challengingfor inexperienced radiologists and a possible pitfall of WB-MRIs.9 Given that not all scanning centres offer WB-MRI images, and the precarious state of oncological patients, care must be taken in standardised reporting of the scans, preferably by experienced radiologists.
CONCLUSION
WB-MRI is evolving into a routine method for evaluating oncology patients for whole-body tumour burden and metastatic disease. With the addition of DWI to a WB-MRI protocol, the characterisation of detected lesions for malignancy and the assessment of treatment response is more optimal. Additionally, with the advent of 3D WB-MRI techniques, higher resolution whole body imaging can be obtained with faster acquisition times and higher resolution, thereby advancing the feasibility of performing WB-MRI for routine clinical practice in oncology patients. To maximise the clinical utility of WB-MRI, efforts to standardise imaging protocols, employ appropriate field strengths, and minimise artefacts are essential. Ultimately, through continued advancements in imaging technology and collaborative efforts to establish standardised guidelines, WB-MRI is poised to become an integral component of oncological imaging in routine clinical practice.