Abstract
Microwave ablation is an effective treatment modality for T1a and selected T1b renal cell carcinoma (RCC). Independent of the type of thermal ablation techniques used, there are important technical considerations for the successful destruction of targeted tumours while minimising complications, including the use of ultrasound for antenna placement and ablation monitoring, immediate post-procedure contrast-enhanced CT for detection of incomplete treatment and early detection of complications, and hydrodissection and hydroureter for prevention of intraprocedural complications. A review of the existing clinical literature demonstrates a low rate of local tumour progression (LTP) following microwave ablation, aswell as a shorter hospitalisation and potentially fewer complications compared to surgery. However, not all patients are candidates for microwave ablation, which can be user-dependent and technically challenging. Overall, microwave ablation is a valuable modality for the minimally invasive treatment of RCC. The purpose of this article is to summarise the literature concerning microwave ablation of RCC with comparisons to other thermal ablation techniques, as well as partial and radical nephrectomy. Technical and clinical considerations are also discussed, with an emphasis on antenna placement, hydrodissection, imaging guidance, and follow-up.
Key Points
1. Thermal ablation is a recommended first-line treatment for the management of small renal masses and is considered a viable treatment alternative for larger renal masses in patients who are not good surgical candidates.
2. In a narrative review of the current literature, microwave ablation is shown to be an excellent choice for the treatment of certain cases of renal cell carcinoma due to minimal invasiveness, preservation of renal function, and excellent oncologic outcomes.
3. Many of the limitations of microwave ablation such as risk of thermal injury to surrounding structures, collecting system, ureteral injuries, and incomplete treatment, can be mitigated with appropriate techniques, including the use of hydrodissection, pyeloperfusion, and real-time monitoring with ultrasound.
INTRODUCTION
Image-guided percutaneous thermal ablation is widely accepted as an alternative to partial nephrectomy in patients with small localised renal cell carcinoma (RCC) due to a lower risk of complications, shorter hospital stays, and disease-specific mortality indistinguishable from surgery. The most recent guidelines from the American Urologic Association (AUA) and the European Association of Urology (EAU) reflect this consensus, with ablation now considered an alternative first-line therapy to surgery.1,2 Additionally, percutaneous ablation may be an acceptable alternative to surgery for larger renal masses in select high-risk surgical patients.3
The choice of thermal ablation technique varies considerably from centre to centre and is largely based on local experience and expertise. The most widely studied thermal ablative modalities with the longest follow-up data are radiofrequency (RF) ablation and cryoablation. Microwave (MW) ablation is a more recent addition and has potential advantages over both of these modalities, including rapid tissue heating, no requirement for grounding pads, no limitations from charring or desiccation, a low risk of post-procedural bleeding, and penetration of all biological tissues.4
The purpose of this article is to review percutaneous MW ablation for the treatment of renal tumours. Topics that will be covered include a discussion on the mechanism of action, particularly as compared to RF and cryoablation, a review of unique technical considerations when treating RCC with MW, and a review of the literature on microwave ablation-related local tumour control, complications, and disease-specific survival. Additionally, there will be a discussion on patient selection procedural and imaging follow-up, and the important role of a multidisciplinary team.
MICROWAVE ABLATION MECHANISM OF ACTION AND A COMPARISON WITH RADIOFREQUENCY AND CRYOABLATION
MW heating is created primarily by the agitation of water molecules by alternating electromagnetic fields at 915 MHz or 2.4 GHz in clinical systems.5 Cell death (tissue ablation) occurs within hours in tissues exposed to temperatures above 42 ℃ and within seconds above 60 ℃.6 Note that boiling occurs in tissue at temperatures above 100 ℃, and thus, cell death can occur even without the formation of visible gas.
MW energy is produced in a controlled amplification system, or generator, and applied to tissue through an antenna that is specifically designed to radiate efficiently in biological tissues. A coaxial cable is used to carry power from the generator to the antenna. The power delivery cable and antenna delivery system contain imperfect conductors and dielectric materials, so some MW power is absorbed in those materials and converted to heat. Active cooling with water or cryogenic gas is employed in most MW ablation systems to mitigate the risk of burns along the antenna or cables. Cryogenic gas cooling can be applied in small-diameter antennas and provide a small freeze zone near the distal tip to ‘stick’ the antenna in place to prevent movement prior to heating.7 Water cooling can be applied with minimal equipment infrastructure, and when it encompasses the entire radiating structure, it may help stabilise antenna performance during the course of MW ablation.8
MW ablation can be performed with a single antenna or an array of antennas. Multiple antennas can be powered either by a single generator, from which power is divided or by multiple individual generators. The synchronous application of MW power using multiple antennas has been shown to create larger and more uniform ablation zone geometries when compared to the sequential application of power by a single antenna.9,10 The details of MW system architecture are beyond the scope of this article, but there are several reviews available on this topic.11-14
MICROWAVE VERSUS RADIOFREQUENCY
Both MW and RF ablation destroy targeted tissue by heating, but the mechanisms of action are different. RF relies on the conduction of an electrical current between the applied electrode and a separate ground pad. The impedance along this pathway can vary from patient to patient. Thus, the amount of current deposited in tissue can also vary resulting in unpredictable heating. During RF ablation, increases in tissue temperatures can cause boiling and charring if unchecked. Subsequent rises in impedance decrease current flow, limiting ablation zones to ~1.0 cm.15 To compensate, RF systems need to either be cooled (limiting tissue temperatures to <100 ℃ to avoid boiling and charring), used multiple times to distribute heat spatially, coupled with a liquid infusion, or heating must be performed slowly and below boiling temperatures.16,17
In contrast, MW heating occurs by an applied electromagnetic field, not an electrical current. As a result, there is no need for grounding pads, and MW energy will propagate through any biological tissues, including those with low water content. The high temperatures associated with MW ablation (>150 ℃ in some cases) reduce the influence of flowing blood and other heat sinks.18 Another difference from RF is tissue contraction, in which heated tissue shrinks around the MW antenna. This effect is correlated to water vaporisation and is, therefore, much more pronounced during MW ablation.19,20 Such tissue contraction is likely a factor in the superior local control seen in many studies comparing MW and RF in various tissue types.5 Finally, the real-time imaging changes observed during thermal ablations, including soft tissue gas and surrounding tissue hypoechogenicity or hypoattenuation on ultrasound and non-contrast CT, respectively, are more predictive of the kill zone for MW ablation than RF.21
MICROWAVE VERSUS CRYOABLATION
Cryoablation involves the percutaneous placement of cryoprobes, which rapidly remove heat from the surrounding tissue.22 One of the major advantages of cryoablation is that tissue freezing can be directly visualised by both CT and ultrasound.23,24 However, an associated pitfall is that the visualised iceball (i.e., the 0 ℃ isotherm) is larger than the lethal zone (the -20 ℃ isotherm).25,26 The lethal zone is unable to be visualised, and therefore, the visible iceball needs to be larger than expected to account for both the lethal isotherm as well as an ablative margin. To obtain larger lethal zones, a large number of cryoprobes and large-diameter probes are often required.27 Tissue ablation is more rapid with MW than cryoablation. After antenna or cryoprobe placement, MW ablations typically last 5– 10 minutes, whereas cryoablation requires multiple freeze-thaw cycles, which are more time-intensive (15–25 min per freeze-thaw cycle, multiple usually required). Finally, cryoablation can be complicated by clinically significant haemorrhage, which may require subsequent embolisation. In an effort to mitigate this risk, some centres perform pre-ablation selective embolisation as a preventative strategy.28,29 The bleeding risk with cryoablation increases with tumour size and with the size and number of probes.30
MICROWAVE ABLATION OF RENAL TUMOURS: SPECIAL CONSIDERATIONS
If and When to Biopsy Renal Masses Scheduled for Ablation
Current AUA guidelines recommend renal mass biopsy prior to ablation as a separate procedure as the preferred approach over biopsy at the time of ablation.31 There are several advantages to this strategy. Up to one-third of small renal masses are benign and may only require surveillance.32 For patients undergoing ablation, a biopsy performed on a separate pre-ablation date is more likely to yield a diagnostic result (with the opportunity for re-biopsy in non-diagnostic cases). A pre-ablation biopsy model avoids prolonged imaging surveillance (often lasting decades) for patients without a definitive cancer diagnosis.33 From a technical standpoint, concurrent biopsy at the time of ablation can make the ablation procedure more complex due to haemorrhage and the introduction of air, which can degrade the acoustic window and obscure visualisation of the ablation target.
Preservation of Renal Parenchyma: Lessons Learned from the Surgical Literature
In addition to the risks of kidney cancer, it is critical to consider the risk of worsening chronic kidney disease (CKD) and the impact on overall survival. Nephron-sparing approaches such as partial nephrectomy are prioritised over radical nephrectomy for T1 tumours and for select T2 tumours because of favourable oncologic outcomes while minimising loss of renal function.34 The development of CKD frequently competes with cancer as a potential cause of mortality in patients treated for RCC, and poor presurgical estimated glomerular filtration rate (eGFR) or a marked drop in eGFR after nephrectomy are associated with increased all-cause mortality.35 In terms of the ideal size of surgical margins, a large meta-analysis concluded that tumour enucleation (1 mm normal renal parenchyma included) was non-inferior to partial nephrectomy (1 cm normal renal parenchyma included) for local and intra-renal recurrence.36 In the absence of definitive literature to the contrary, this concept of narrow but negative margins should be extended to the ablation of RCC. Positive margins after surgery or incomplete ablation are associated with patient anxiety and worse recurrence-free survival.37,38 Compared to RF and cryoablation, MW creates precise ablation zones with a narrow zone of partial necrosis between treated and untreated tissue that may help minimise unwanted destruction of normal renal parenchyma.21,39
Injury to the Collecting System and Ureter with Microwave Ablation
Injuries to the collecting system, renal pelvis, and ureter have been reported with all thermal ablation modalities but are likely higher with heat-based modalities, given the relative preservation of collagenous structures with cryoablation.40,41 Urothelial injuries from MW can be severe due to the high temperatures and power applied to the tissue. Injuries to isolated calyces adjacent to the targeted tumour are common and generally of no consequence. However, if a calyceal injury and a proximal infundibular stricture are concomitantly created, a patent probe track can lead to urinoma formation. This complication can be avoided using a tangential probe path (Figure 1), avoiding direct collecting system punctures, and forgoing track cautery.42 Rarely, more severe injuries involving the renal pelvis or ureter can result in ipsilateral kidney loss. The mechanism of these injuries is likely due to high applied powers, generally seen with multiple MW antennas, which can create steam within the collecting system, causing urothelial injury and scarring.40 This complication can generally be avoided by careful attention to probe number, power, and ablation time, as well as real-time monitoring of the ablation with ultrasound. Retrograde pyeloperfusion is another protective measure that has been shown to reduce urothelial injuries during cryoablation and, anecdotally, is also effective for use with MW.43 Patients should be well informed of the small risk of collecting system injury and impact on future renal function.
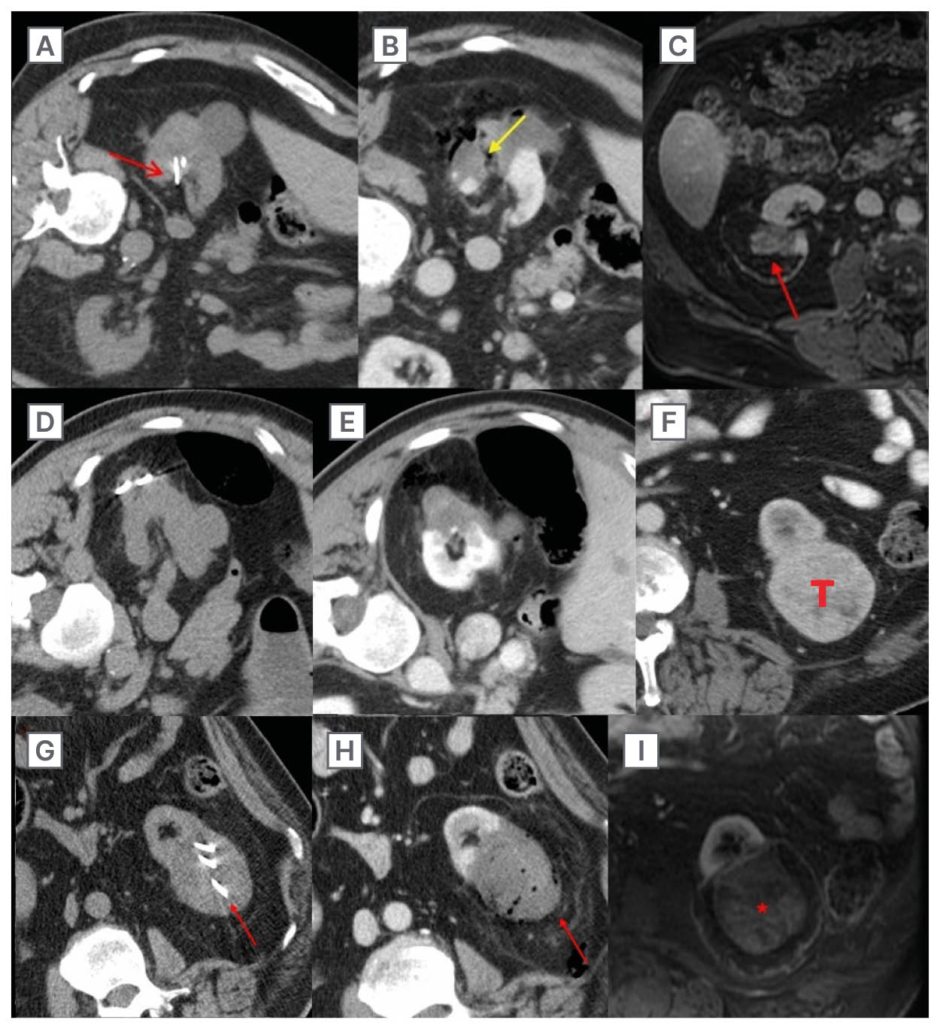
Figure 1: T1a/T1b renal cell carcinoma probe placement.
Potential consequences of incorrect antenna positioning and proper antenna positioning in MW ablation of T1a/T1b RCC.
A–C) T1a RCC treated with MW ablation with incorrect probe placement. A) Non-contrast CT performed during probe placement. Note the deep placement of the probes into the collecting system (red arrow). B) Immediate post-treatment contrast-enhanced CT. Track cautery (not recommended) results in a patent fistulous connection (yellow arrow) between the collecting system and perirenal space. C) 24-month follow-up contrast-enhanced MRI demonstrating an asymptomatic urinoma (red arrow).
D–E) T1a RCC (separate patient) treated with MW ablation with the recommended tangential probe placement avoiding collecting system puncture. D) Non-contrast CT performed during probe placement demonstrates tangential placement of antennas avoiding the collecting system. E) Immediate post-treatment contrast- enhanced CT with adequate treatment of the tumour, a small margin, and no evidence of complications.
F–I) T1b 6.3 cm T1 RCC treated with MW ablation with multiple probes. F) Contrast-enhanced CT performed pre-ablation. G) Non-contrast CT during probe placement (red arrow). H) Immediate post-treatment contrast-enhanced CT demonstrates a lack of tumour enhancement and no evidence of post-procedure complications. I) 15-month follow-up contrast-enhanced MRI shows a continued lack of enhancement of the tumour (*).
MW: microwave; RCC: renal cell carnioma.
MICROWAVE ABLATION TECHNIQUE
System and Setting
Renal MW ablation technique varies by institution. At the authors’ institution, renal MW ablation is performed by a multidisciplinary team of abdominal/interventional radiologists and urologic oncologists. Most commonly, patients are treated in the contralateral decubitus position, although oblique or prone positioning may be used. Ultrasound is preferred for guiding antenna placement. Rarely, if the tumour is not well visualised by ultrasound, CT fluoroscopy, or a CT-based needle navigation system (Imactis CT-Navigation, GE Healthcare, Chicago, Illinois, USA) is utilised. Non-contrast CT is used to confirm accurate antenna placement and spacing prior to energy delivery. MW is performed with a multiprobe, gas-cooled, 2.45 GHz system with synchronous energy delivery (Neuwave Medical, Johnson & Johnson, New Brunswick, New Jersey, USA). Ablations are performed under general anaesthesia with high-frequency jet ventilation (HFJV), which is known to decrease kidney motion and improve targeting and monitoring.44
Probe Number, Antenna Placement, and Energy Delivery
One to three MW antennas are used in each case at the discretion of the operator. In general, tumours ≤2 cm in diameter can be treated with a single antenna, tumours 2–4 cm are treated with two antennas, and tumours >4 cm require three antennas. When compared with exophytic tumours of a similar size, endophytic tumours may require more antennas due to greater heat sink from surrounding renal parenchyma compared to perirenal fat. It is essential to understand the expected size, shape, and location of the ablation zone relative to the antenna tip(s) for a given set of ablation parameters (input power, ablation time). However, manufacturer-provided data in “lookup tables,” obtained in ex vivo tissue, may not accurately reflect results in clinical cases. These differences can be pronounced for tumours in highly perfused tissue, such as endophytic renal tumours.45
Antennas are ideally placed tangential to the tumour, avoiding collecting system puncture to avoid urinomas and collecting system injuries (Figure 1).42
Ablations are usually performed using maximum energy delivery (power), typically 65 watts for 5 minutes, and adjusted based on real-time ultrasound monitoring. Power is decreased if the ablation zone has grown to an adequate size and there is concern for overtreatment or injury to critical structures. If there is inadequate tumour coverage based on real-time monitoring, additional time is added as MW ablation zones can continue to grow for up to 15 minutes.46 Proximity to vulnerable extrarenal structures, such as the bowel, pancreas, or body wall, can generally be managed with hydrodissection, discussed in greater detail below, using 2% iohexol in normal saline.47 Following ablation, the antennas are removed without cautery to avoid creating coagulated tracts that may lead to urinomas. An immediate contrast-enhanced diagnostic CT is performed in the interventional suite to determine adequacy of treatment, with retreatment if necessary, and to assess for early complications such as haemorrhage.48
Careful patient selection and MW technique are necessary when considering treatment of larger T1b (4–7 cm) renal tumours. Compared to T1a tumours, T1b tumors require a great number of antennas, longer treatment times, and higher power, which can increase the probability of complications and the risk of incomplete treatment compared to T1a tumours.3,49 At the authors’ institution, the decision to treat a T1b tumour with MW is a multidisciplinary one, with surgery as the preferred option for these patients. A primary consideration for favouring MW over surgical treatment are patients who are high risk surgical candidates, usually due to significant comorbidities. An additional consideration that can favour MW over surgery is the primacy of preserving renal parenchyma, either due to prior nephrectomies or the expectation that future nephrectomies will be required, as in the case of patients with genetic renal tumor syndromes. Anatomic factors that facilitate the use of MW ablation for the treatment of T1b tumours are tumours that are at least partially exophytic, those removed from the renal hilum, and those in locations where hydrodissection is feasible. Treatment of T1b tumours is virtually always accompanied by hydrodissection to decrease the potential for damage to non-targeted structures (bowel, pancreas, ureter), and there is a low barrier to the use of pyeloperfusion to protect the collecting system. During and after probe placement and during application of MW energy, liberal use of both ultrasound and CT monitoring is recommended to both assure coverage of the tumour and to monitor for complications in a similar manner as with T1a tumours.
Tumour Location: Implications for Microwave
Certain tumour locations increase treatment complexity, complications rates, and risk of treatment failure.50 In general, tumours that are peripheral and located in the lateral or posterior kidney are at decreased risk of injury to adjacent structures with MW. Endophytic lesions can be more challenging to visualise than exophytic lesions and risk collecting system injury. Medial lesions abutting the psoas muscle risk genitofemoral nerve injury.24 Anterior lesions risk bowel or pancreatic injuries. Upper pole lesions can be challenging due to proximity to the diaphragm and intervening pleura, and are more likely to require use of advanced imaging techniques for targeting and monitoring including CT fluoroscopy, CT/US fusion, and electromagnetic navigation for off-axial approaches. Lastly, particular attention needs to be paid to anterior-inferior tumours where ureteral injury becomes possible. In most cases, injury to non-targeted structures can be mitigated with adequate hydrodissection, which should be used liberally when in doubt.51
Ultrasound versus CT Monitoring
Ultrasound monitoring is favoured as it can continuously monitor the growing ablation zone and is more predictive of the pathologic zone of necrosis than non-contrast CT.21 However, if visualisation becomes limited by gas or intervening structures, intermittent CT is recommended. In the rare cases when CT fluoroscopy is utilised for antenna placement, ultrasound is still used to monitor the ablation, given its superior visualisation of the evolving treatment changes in real-time. Ultrasound is particularly effective for multiplanar monitoring, as proximity to the bowel may not be fully appreciated on axial CT fluoroscopy. Ultrasound also allows for real-time monitoring of hydrodissection to determine whether additional fluid is necessary to maintain adequate displacement of critical structures during treatment.
Hydrodissection
Hydrodissection is frequently used to protect adjacent structures, such as the bowel and body wall, from thermal injury. Normal saline (0.9% NS) with 2% iohexol injected through a purpose-designed blunt-tipped needle (Gangi Hydroguard, AprioMed, Uppsala, Sweden) can be easily distinguished from bowel, solid organs, and muscle without causing beam-hardening artefact (Figure 2A).47 Care must be taken to avoid injecting gas bubbles, which limit ultrasound visualisation during probe placement and monitoring.
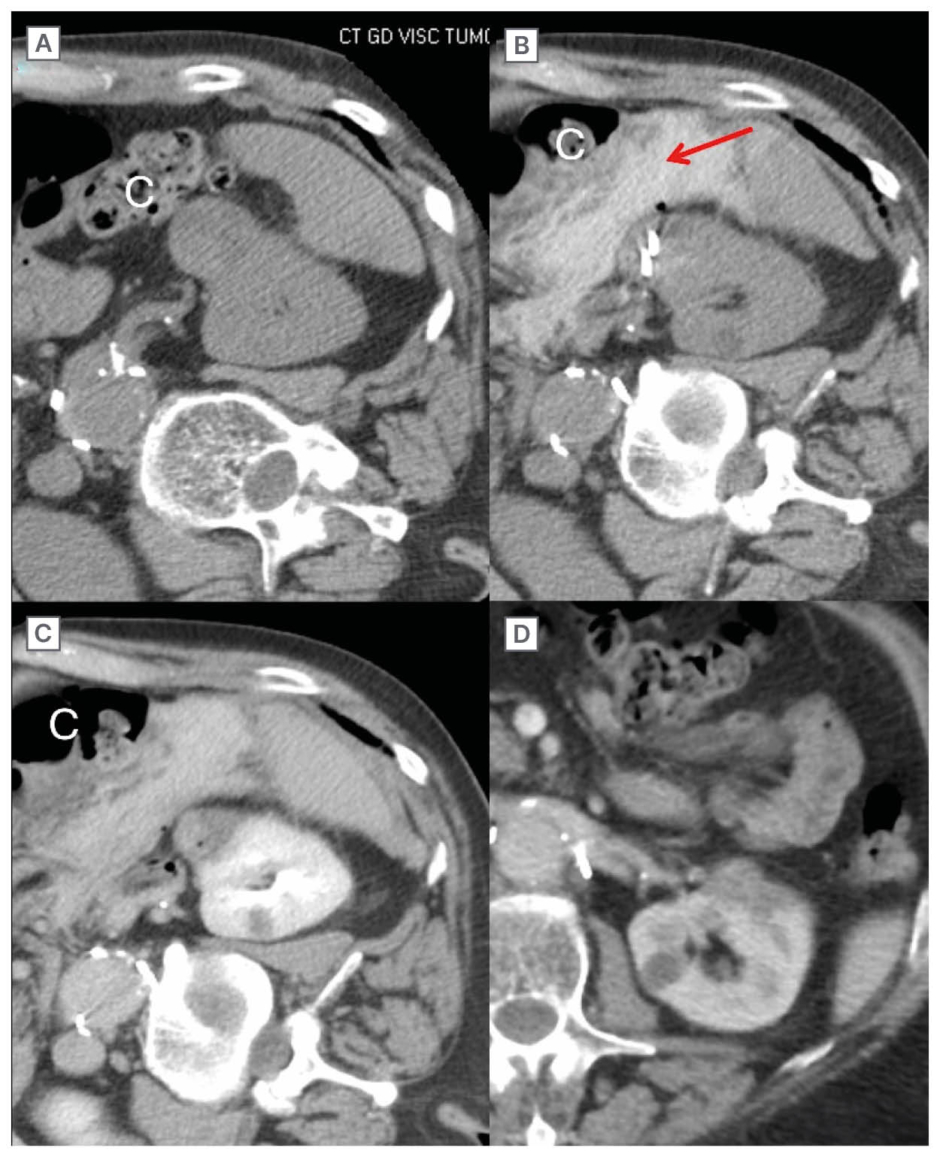
Figure 2A: Hydrodissection to displace colon and small bowel.
A) Non-contrast CT demonstrates close contact of the tumour with colon, which would likely be injured without hydrodissection. B) Non-contrast CT after probe placement and administration of hydrodissection fluid (red arrow). The colon is now displaced away from the tumour, and it is safe to proceed with ablation. C) Immediate post-treatment contrast-enhanced CT. The probes have been removed, and the displacement between the tumour and colon remains. Note that the tumour has substantially contracted, which is typical of MW. D) 8-month follow-up contrast-enhanced CT. The tumour continues to shrink, the hydrodissection fluid is resorbed, and there is no evidence of bowel injury.
C: colon; MW: microwave.
Nephroureteral Stents and Retrograde Pyeloperfusion
Ureteral injury from heat can potentially be mitigated using indwelling ureteral stents or placement of an internal-external retrograde catheter for pyeloperfusion with cooled saline.24 There is no consensus as to the most effective method to prevent urothelial injuries, although pyeloperfusion is highly effective for this purpose during cryoablation (Figure 2B).43
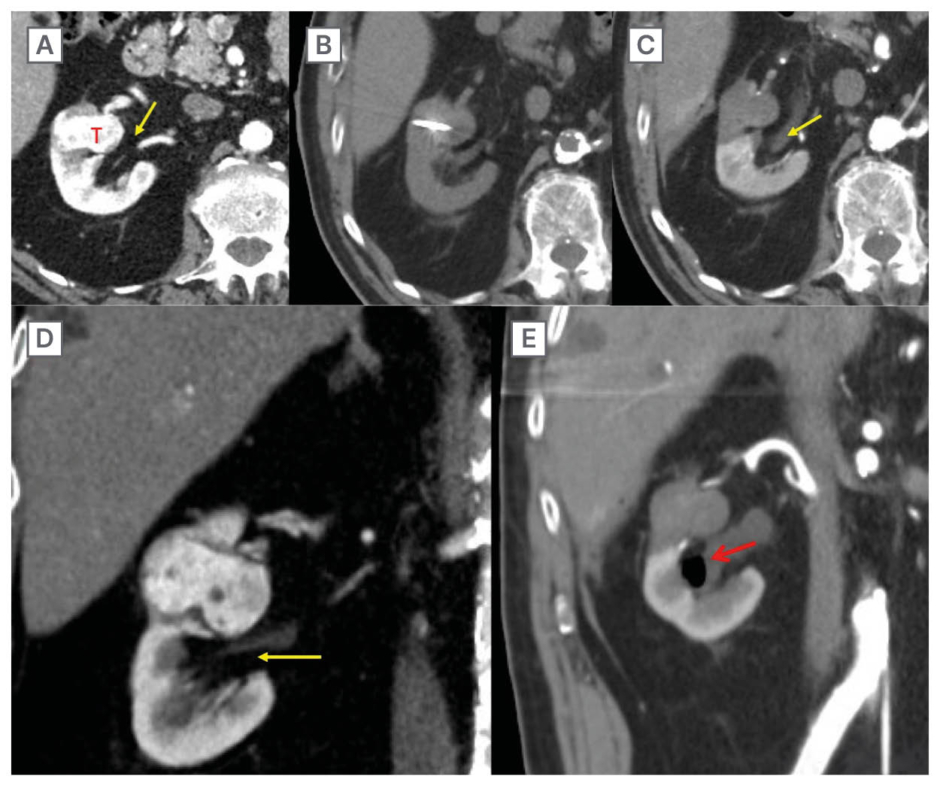
Figure 2B: Retrograde pyeloperfusion.
T1a RCC (3.5 cm) in close proximity to the renal pelvis, which is at risk of injury. A, D) Contrast-enhanced CT performed pre-ablation in the axial (A) and coronal (D) planes. Note the close proximity of the tumour (T) and renal pelvis (yellow arrow). B) Non-contrast CT demonstrating probe placement. C, E) Immediate post-treatment contrast-enhanced CT (C axial, E coronal) demonstrates lack of tumour enhancement. Note the distention of the renal pelvis (yellow arrow) by the retrograde injection of cooled saline through a ureteral stent (not shown). A small amount of air was injected with the fluid and is present in the renal pelvis (red arrow). The renal pelvis and collecting system were not injured despite the close proximity of the ablation zone in this central tumour.
Post Ablation Imaging Follow-Up
Follow-up imaging with contrast-enhanced CT or MRI is important to ensure early detection of residual tumour and to evaluate for local recurrence. Preferred imaging modality and interval vary by ablation modality, operator preference, and institutional resources. In general, contrast-enhanced CT should be performed immediately post-treatment to ensure adequate tumour coverage with immediate retreatment if necessary.48 On post-contrast imaging, the ablation zone roughly correlates with the zone of altered signal intensity or decreased perfusion, with areas of viable but malperfused tissue occasionally seen peripheral to the ablation zone. Residual viable tumour or recurrence typically manifests as a nodular or mass-like area of enhancement with imaging characteristics similar to the treated tumour. The interval between ablation and first follow-up should be guided by the histologic grade of the tumour, reinforcing the critical nature of biopsy prior to treatment, but the first follow-up imaging examination can safely be performed at 6 months for nearly every tumour treated with ablation.52
LENGTH OF HOSPITALISATION AND PROCEDURE TIME, QUALITY-OF-LIFE IMPACT, AND COST-BENEFIT
One of the most significant advantages of MW ablation, and all percutaneous ablation modalities, is a significantly shorter length of hospitalisation compared to surgical interventions. At the reference institution, most patients are discharged either the same day or the following morning. The median hospitalisation length for patients treated with MW is between 1.8–3.6 days shorter compared to those treated with surgery.3,53,54 Although there is no significant difference in hospitalisation length between thermal ablation methods, the mean procedure time for MW ablation is significantly shorter than RF ablation or cryoablation.55,56 Quality-of-life measures after each of the percutaneous ablation techniques demonstrate minimal impact and a short-term advantage versus partial nephrectomy, likely due to the less invasive nature of the procedure. However, the data in this area is not robust, and there are a paucity of studies directly comparing MW to other percutaneous ablation modalities as well as partial nephrectomy.57,58 Cost-effectiveness data on MW versus partial nephrectomy is likewise sparse, but available studies suggest that MW may be competitive with PN based on total life years, quality-adjusted-life-years, and lifetime costs.59,60
COMPLICATIONS AND PRESERVATION OF RENAL FUNCTION
Treatment of RCC with MW ablation is Cassociated with a low and predominantly minor (Clavien-Dindo Grade I-II or CIRSE I) complication rate with a similar safety profile to other thermal ablation methods.42,56,61 Partial nephrectomy may be associated with a higher but not significant major complication rate.53 MW appears to effectively preserve renal function, with no significant change in the estimated glomerular filtration rate post-procedure.61 Comparative studies suggest that MW is less likely to cause acute kidney injury and preserves renal function more effectively than laparoscopic partial nephrectomy but is similar to RF and cryoablation.53,62,63 For T1b tumours, eGFR decline is comparable between MW ablation and partial nephrectomy. Both partial nephrectomy and MW preserve renal function to a higher degree than radical nephrectomy.3 In many series, although patients treated with MW were older and had more comorbidities, they did not have a higher risk of serious complications compared to partial or radical nephrectomy. However, high-grade complications after MW are more common in T1b tumours than with smaller renal masses.3
TECHNICAL SUCCESS/INCOMPLETE TREATMENT
Technical success is defined as a complete treatment of the targeted tumour on immediate post-procedure imaging.64 MW ablation shows technical efficacy comparable to RF ablation and cryoablation, with success rates surpassing 94% for the complete treatment of T1a lesions.56,63,65 Increasing tumour size can be associated with lower rates of technical success. Although high efficacy has been observed for the treatment of T1b (>4 to <7 cm) renal tumours at experienced centres, this experience is not universal, with others reporting higher rates of incomplete treatment with larger lesions requiring repeat ablation.3,49
LOCAL TUMOUR PROGRESSION
Local tumour progression (LTP) is defined as the emergence of a tumour at the edges of an ablation zone on follow-up imaging after a technically successful treatment.64 MW ablation is associated with a low local tumour progression rate, ranging from 0–7.5% when treating small renal cell carcinomas.49,56,61,63,66 Comparative studies show that MW ablation achieves similar or lower LTP rates compared to other ablative techniques, such as RF ablation and cryoablation, while maintaining high technical success (Table 1).49,55,63 A meta-analysis further supports these findings, indicating that MW is associated with a significantly lower 1-year local tumour progression rate compared to cryoablation.71 Retrospective studies comparing MW ablation with partial nephrectomy report no significant differences in LTP between the modalities, highlighting MW ablation as a less invasive but effective alternative for managing small RCC in appropriately selected patients.3,53 These studies must be interpreted in the context of their observational nature and patient selection biases.
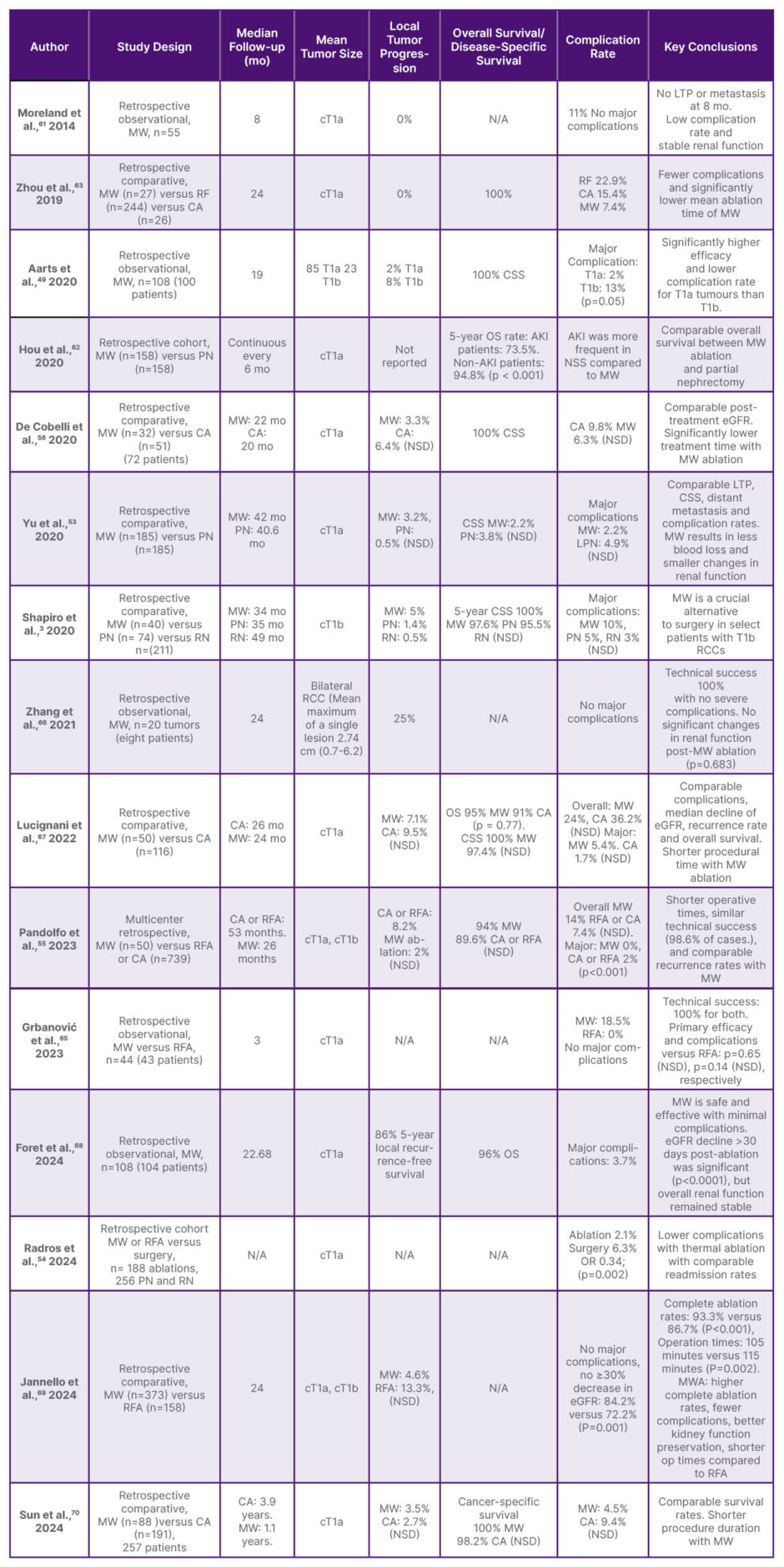
Table 1: Literature summary for MW ablation of renal cell carcinoma.
Major complications defined as Clavien-Dindo Grade ≥3.
AKI: acute kidney injury; CA: cryoablation; CSS: cancer-specific survival; MW: microwave; NSD: no significant difference; OS: overall survival; PN: partial nephrectomy; RCC: renal cell carcinoma; RFA: radiofrequency ablation; RN: radical nephrectomy.
OVERALL SURVIVAL
MW ablation offers similar overall survival rates compared to RF and cryoablation.71 A single meta-analysis comparing MW ablation and cryoablation concludes that there is no significant difference in overall survival rates between MW ablation and cryoablation.63 Several studies demonstrate worse overall survival for MW ablation versus partial nephrectomy but comparable cancer-specific survival.3,53 It is important to note that disease-specific survival or cancer-specific survival is a better metric to compare ablation to surgery, as overall survival may be confounded by non-cancer-related factors, such as a higher Charlson comorbidity index in specific patient groups.53 This is especially important since ablation is more commonly utilised in older patients with increased comorbidities.72
LIMITATIONS/FUTURE RESEARCH NEEDS
Although studies with high levels of evidence are included in this review, the observational and retrospective nature of the bulk of the available clinical data presents a limitation. Additionally, available studies varied in follow-up duration, had an unequal distribution of comparison groups, and were conducted in centres with different levels of experience.3 An important limitation in comparing percutaneous ablation to surgery is the inherently different patient populations usually treated by each group. For example, as noted above, most ablation cohorts are older and more morbid than surgical patients. Future randomised controlled trials and observational studies with propensity matching of the different cohorts that account for these and other variables will be important steps towards addressing these limitations.
CONCLUSION
Percutaneous MW ablation is an effective alternative to partial or radical nephrectomy for T1a renal tumours, particularly for patients who prefer a less invasive option or who are high-risk surgical candidates that still require tumour control. For T1b renal tumours, surgical excision is the preferred approach; however, percutaneous ablation can be considered for patients with substantial comorbidities or contraindications to surgery at experienced institutions. Observational studies of MW ablation demonstrate comparable local tumour progression and cancer-specific survival rates compared to partial nephrectomy, shorter hospitalisation lengths, lower complication rates, and similar renal functional preservation. However, MW ablation remains user- and technique-dependent, and local expertise is critical. MW offers several advantages compared to other thermal ablation modalities, including speed, effectiveness in destroying targeted tumours, accurate real-time intraprocedural monitoring with ultrasound, and single or multiple applicator use. MW ablation requires caution when treating tumours in the central kidney or adjacent to the renal pelvis and ureter due to the risk of strictures and urinomas. Important considerations for a successful MW ablation programme for the treatment of RCC include multidisciplinary input, attention to anaesthesia technique with consideration of the use of jet ventilation, intraprocedural monitoring with ultrasound, placement of antennas parallel and outside of the collecting system, careful hydrodissection technique, retrograde pyeloperfusion for ureteral protection, and systematic longitudinal imaging follow-up.