Abstract
Lung cancer has a devastating global impact, with diagnosis of more than 2 million new cases annually, and poor long-term survival. Recently, the landscape of lung cancer diagnosis, staging, and treatment has changed profoundly, with further developments on the horizon.
It has become of increasing importance to comprehensively characterise lung tumour tissue. Minimally invasive diagnostic modalities, including standard bronchoscopy and radial probe endobronchial ultrasound (EBUS), enable adequate tissue sampling for tumour subtyping. Sophisticated electromagnetic navigation software and novel biopsy procedures have allowed for sampling of even very peripheral tumours, in the hands of experienced bronchoscopists. Linear EBUS is now widely used for simultaneous diagnosis and cancer staging, reducing time to treatment initiation and effectively replacing invasive mediastinoscopy. Liquid biopsy is an emerging noninvasive technology with potential for diagnosis, prediction of tumour response, and detection of resistance-related gene mutations.
Significant advancements in our understanding of the immunologic and oncogenic processes involved with lung cancer biology have helped revolutionise management. Whilst chemotherapy remains a therapeutic cornerstone for many, evolving evidence supports a personalised approach, particularly in advanced disease. Specific inhibitors targeting driver mutations and key immunological pathways confer survival benefits in metastatic lung cancer, with emerging data in early stage disease.
In this review, lung cancer histological subtypes are discussed, with a focus on non-small cell lung cancer, along with current and evolving approaches to diagnosis and staging. Therapeutic options in the era of precision medicine will also be considered within the context of targetable oncogenic driver mutations and the growing field of immuno-oncology.
INTRODUCTION
Lung cancer has a devastating global impact. Claiming over 2 million lives in 2018, it is the world’s leading cause of cancer-related morbidity and mortality.1 Five-year survival rates are estimated to be less than 20%. Until recently, treatment options have been limited to surgery for early stage disease, and systemic chemotherapy for unresectable, locally-advanced, and metastatic disease. Recent advances in our understanding of molecular pathobiology of lung cancer have paved the way towards a personalised approach to treatment. The discovery of specific targetable mutations and understanding of the pivotal role of immunosurveillance in suppressing malignant growth have allowed for the development of innovative therapeutic strategies. This review will broadly cover updates in the personalised management of lung cancer, particularly the non-small cell subtype, including the importance of accurate histological characterisation through to novel treatment options guided by targetable oncogenic driver mutations, the immunological influences on tumour growth, and the emerging technologies for precise molecular profiling of individual cancers.
THE IMPORTANCE OF DISTINGUISHING HISTOLOGICAL SUBTYPE IN LUNG CANCER
Lung cancer can be subdivided into two major histological subtypes: non-small cell lung cancer (NSCLC), accounting for approximately 85% of cases, and small cell lung cancer, in the remaining 15%.2 NSCLC can be further subclassified into adenocarcinoma, squamous cell carcinoma, and large cell carcinoma. Tumour subtype can be determined by morphological features on cytology and histopathology, as well as immunohistochemical staining. For example, TTF1, napsin A, and cytokeratin 7 positivity favour a diagnosis of adenocarcinoma, whilst positivity for p40, p63, and cytokeratins 5 and 6 are suggestive of squamous cell carcinoma.3
Treatment, staging, and outcomes can be markedly different between small-cell and non-small cell lung tumours, with small cell cancers generally behaving more aggressively and conferring poorer prognosis. Historically, distinguishing the non-small cell tumours by subtype had minimal impact on management until the discovery that histology influenced therapeutic outcomes was made. Specifically, treatment of adenocarcinoma with bevacizumab, a humanised monoclonal antibody targeting VEGF, improved both progression free and overall survival in adenocarcinoma but increased the risk of catastrophic pulmonary haemorrhage in patients with squamous cell carcinomas.4 More recently, the discovery of specific oncogene mutations in certain tumour subtypes has further emphasised the importance of detailed tumour characterisation. Specific driver mutations have been identified in many lung adenocarcinomas (less frequently, however, in squamous cell carcinomas), and have been associated with cell proliferation, tumour growth, and survival. These mutations are usually mutually exclusive of each other and result in the transformation of noncancerous cells towards malignant cell lines, resistant to the usual regulatory processes. Targeting the protein products of these mutations with specific inhibitors can have a major effect on susceptible tumours, allowing for a precision medicine approach to treatment.
ESTABLISHING A DIAGNOSIS
In order to inform appropriate management, sufficient quantities of tissue must be obtained to identify the precise histological diagnosis (Table 1).5 Lung tumours can be biopsied percutaneously using radiologic guidance with CT or endobronchial ultrasound (EBUS), with either direct bronchoscopic sampling of the primary tumour or indirect sampling of involved thoracic lymph nodes.
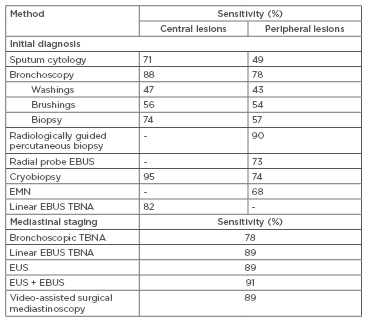
Table 1: Diagnostic and staging methods in lung cancer.
Adapted from McLean et al.5
EBUS: endobronchial ultrasound; EMN: electromagnetic navigation; EUS: endoscopic ultrasound; TBNA: transbronchial needle biopsy.
Due to the rising prevalence of peripheral lung lesions, percutaneous fine-needle and core biopsies with CT guidance have become increasingly utilised, with a pooled sensitivity of up to 90%.6 Biopsies of lesions under 1.5 cm are less likely to be diagnostic, with a sensitivity of approximately 70%.7 More centrally located tumours and concomitant emphysema are associated with a higher risk of pneumothorax, with one study reporting rates of up to 27%.8 Fibreoptic bronchoscopy performed under conscious sedation facilitates a number of different diagnostic approaches depending on the tumour location. The diagnostic rate for central tumours with use of bronchoscopic forceps is reportedly 65–82%, increasing up to 88% if combined with bronchial brushings and washings for cytology assessment.6,9 However, for peripheral lesions that cannot be directly viewed, the sensitivity of diagnostic bronchoscopy has been shown to be as low as 14%, particularly if the lesion is less than 2 cm in size.10 Another bronchoscopic approach for targeting peripheral lesions is with radial probe EBUS, which utilises an ultrasound, enabling 360-degree imaging of surrounding structures. Peripheral lesions can be localised and targeted for transbronchial needle aspiration (TBNA) biopsy. In a recent meta-analysis, pooled diagnostic sensitivity was 73%, with a pneumothorax rate of only 1%.11 Although diagnostic yield of radial-EBUS is lower than transthoracic percutaneous biopsy, the advantage of this approach is a significantly reduced pneumothorax risk.7 Importantly, whilst it may confirm the presence of a target lesion, radial-EBUS does not itself provide a means of navigating to the lesion of interest. Combining radial-EBUS with highly specialised electromagnetic navigation (EMN) technology allows real-time navigation to the target lesion when mapped against a contemporary CT image. In a small randomised controlled trial, Eberhardt et al.12 showed that combining EMN with radial-EBUS significantly improved diagnostic yield to 88%, compared to either radial EBUS (69%) or EMN-standard bronchoscopy (59%) alone, independent of lesion size and lobar distribution.
STAGING THE MEDIASTINUM
Staging of NSCLC (I–IV) is important for determining treatment and prognosis, and requires evaluation of tumour size, lymph node involvement, and presence of metastatic disease, following the International Association for the Study of Lung Cancer (IASLC) TNM staging guidelines.13 Whole-body PET is a sensitive imaging tool for staging NSCLC, particularly when integrated with CT. PET-CT provides accurate assessment of mediastinal disease, helping to guide treatment decisions in patients with NSCLC. Linear or convex probe EBUS with TBNA is the standard diagnostic procedure for patients with radiological PET-avid nodal disease or central primary tumours adjacent to airways.14 It is a minimally invasive procedure with few complications (<1%), even in the elderly population.15 The convex-probe EBUS-TBNA is advantageous over other methods, allowing simultaneous diagnosis and staging, hence reducing time to treatment. In a randomised control trial comparing EBUS with conventional diagnosis and staging, those undergoing EBUS had reduction in median time to treatment decision by >50% (14 versus 29 days, hazard ratio [HR]:1.98, p<0.0001).16
False negative rates of PET-CT can be as high as 25%, and so EBUS-TBNA is also recommended in those who have hilar lymphadenopathy and central tumours, irrespective of mediastinal node PET-avidity.17 Endoscopic ultrasound with TBNA may be a reasonable alternative for lymph node stations that cannot be accessed bronchoscopically.
TREATMENT IN THE ERA OF PRECISION MEDICINE: TARGETING DRIVER MUTATIONS
For decades, cytotoxic chemotherapy has been the cornerstone of management for all but early-stage NSCLC (Table 2).18 The recognition of specific somatic ‘driver’ mutations in NSCLC has transformed both the treatment and outcomes for patients with advanced-stage lung cancer. These mutations occur in oncogenes and tumour suppressor genes, resulting in unregulated cell proliferation and tumour survival. The frequencies of identifiable mutations in lung adenocarcinomas are shown in Figure 1A.19 Targeting these mutated proteins with specific inhibitors has led to a paradigm shift in cancer therapeutics. Agents targeting mutations in EGFR, ALK, ROS1, and BRAF proto-oncogenes have been approved in NSCLC. Specific therapies for the other driver mutations are under development.
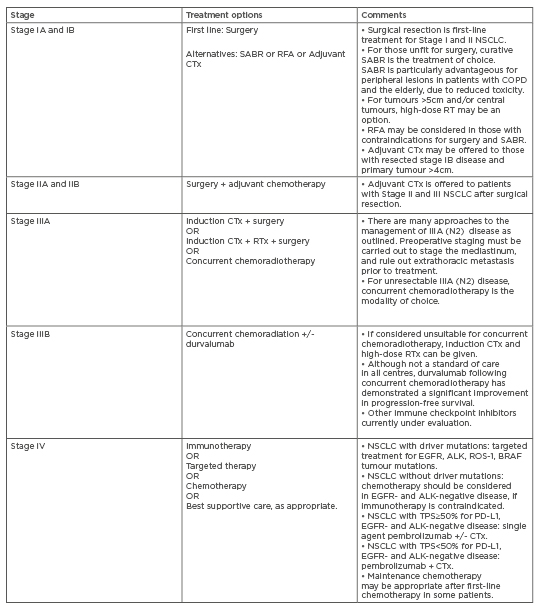
Table 2: Treatment options for Non-Small Cell Lung Cancer.
Adapted from Postmus et al.18
ALK: anaplastic lymphoma kinase; CTx: chemotherapy; EGFR: epidermal growth factor receptor; NSCLC: non-small cell lung cancer; PD-1PD-L1 : programmed death-1/ programmed death-ligand 1; RFA: radiofrequency ablation; RTx: radiotherapy; SABR: stereotactic ablative body radiotherapy; TPS: tumour proportion score.
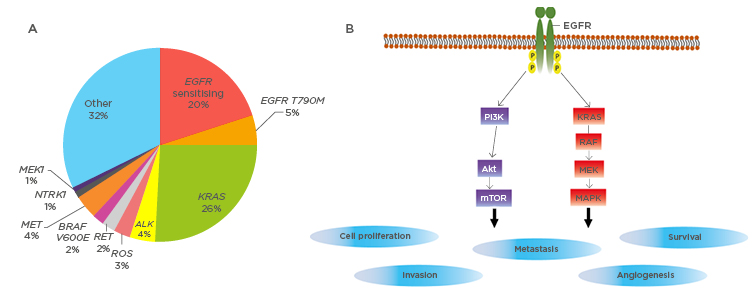
Figure 1: Driver mutations in lung adenocarcinomas.
A) Frequencies of identifiable oncogene driver mutation in non-small cell lung cancers. Adapted from Jordan et al.19
B) The molecular pathogenesis of EGFR sensitising mutations. EGFR mutations lead to ligand-independent activation of downstream signalling pathways, leading to cellular proliferation and survival. Akt: Protein kinase B; E: glutamic acid; EGFR: epithelial growth factor receptor; M: methionine; MEK: mitogen-activated protein/extracellular signal regulated kinase; mTOR: mammalian target of rapamycin; RAF: rapidly accelerated fibrosarcoma; ROS: reactive oxygen species; T: threonine; V: valine.
EGFR Mutations
EGFR mutations were first described in 2004.20 They occur in 10–35% of lung adenocarcinomas, with higher frequency in east Asian populations and in younger females with no previous smoking history.21 The net result of these mutations is constitutive activation of EGFR with stimulation of proliferative signalling pathways (Figure 1B).22 There are now three generations of tyrosine kinase inhibitors (TKI) that target the mutated EGFR. These include first generation erlotinib and gefitinib, second generation afatinib and dacomitinib, and third generation osimertinib. Their efficacy has been established in 13 Phase III randomised controlled trials, clearly highlighting the role of EGFR-TKI as first line treatment in EGFR-mutated Stage IIIB and Stage IV NSCLC.23 The role of EGFR-TKI in the adjuvant setting in Stage II and Stage IIIA disease, however, is less certain. The ADJUVANT/CTONG1104 trial randomised 222 patients with completely resected, EGFR-positive Stage II-IIIA (with N1-N2 nodal involvement) to receive either gefitinib or vinorelbine plus cisplatin. There was a significant improvement in median disease-free survival in the gefitinib arm in comparison to the standard platinum-based chemotherapy arm, (28.7 versus 18.0 months, HR:0.60, p=0.0054).24
Most tumours will develop resistance to these agents even where there is initial good response. The most common resistance mechanism is the T790M mutation.25 Given that second generation TKI have limited ability to overcome T790M resistance, third generation TKI osimertinib was developed as a mutant-sensitive therapy. The AURA3 study included patients with progression on first generation TKI, showing improved overall tumour response rates and progression free survival (PFS) in those randomised to osimertinib, compared to standard platinum-based chemotherapy.26 Further third generation EGFR-TKI are under development.
ALK/ROS1 Mutations
Less common targetable mutations include the ALK gene rearrangements, which result in a chimeric protein (EML4-ALK) with constitutive ligand-independent tyrosine kinase activity.27 Crizotinib has been developed as an agent with specific activity against the chimeric EML4-ALK protein. The PROFILE 1014 study, including patients with ALK rearrangements, demonstrated significant improvements in median PFS and objective response rates for crizotinib versus standard first-line chemotherapy.28 Studies have also demonstrated efficacy in the second line setting, in comparison to standard chemotherapy.29
Newer generation ALK-TKI, including ceritinib, alectanib, and lorlatinib, have been developed to treat tumours with acquired resistance to crizotinib.30 The ASCEND-5 trial revealed superiority of ceritinib over single agent chemotherapy in crizotinib-resistant patients, with significant improvement in median PFS.31 As crizotinib has limited central nervous system (CNS) penetration, a common mode of disease progression is through new brain metastases, necessitating vigilant CNS surveillance.32 Second and third generation ALK inhibitors have improved CNS penetrance with evidence supporting superior outcomes.33 The ALEX study compared alectinib with crizotinib as first line therapy in patients with ALK gene rearrangements. Alectanib was associated with longer median PFS and time to CNS progression.34
ROS1 gene rearrangements account for 1–2% of NSCLC and are more commonly found in young patients with minimal tobacco exposure and with adenocarcinoma histology.35 Crizotinib and other ALK-TKI have shown activity against NSCLC harbouring ROS1 rearrangements because of their structural homology to the ALK protein. In a Phase II study of 127 patients with this oncogene, crizotinib led to objective response rates of 71.7% and median PFS of 15.9 months.36 Specific ROS1 inhibitors are being tested in early-phase trials.
KRAS Mutations
The KRAS mutation is the most common oncogenic driver mutation, occurring in 25–34% adenocarcinomas and 3–6% squamous carcinomas, particularly in smokers of non-Asian ethnicity.37,38 To date, treatment strategies for KRAS-mutant lung cancer have been disappointing. Despite increasing understanding of the molecular biology of these mutations, there are no current specific therapies. Recommended treatment is similar to that of NSCLC without identifiable driver mutations.39
BRAF Mutations
BRAF mutations are found in many cancer cell lines, the prototypical example being melanoma.40 The most common BRAF mutation is the V600E mutation and is observed in 1–2% of lung adenocarcinomas, particularly in patients with a significant tobacco smoking history.41 Following on from experience in melanoma populations, the Phase II BRF113928 trial investigated the combination of BRAF inhibitor dabrafenib and MEK inhibitor trametinib in BRAF-positive NSCLC. Given the rarity of this mutation, the study was small and open label in design. In 36 treatment-naïve patients with metastatic BRAF V600E-mutant lung cancer, combination therapy led to complete or partial response in 23 of the patients (64%).42 These findings have led to regulatory approval of this combination in the BRAF V6003-mutant patient group.
IMMUNOTHERAPY IN THE ERA OF PRECISION MEDICINE
Despite recent advances in the understanding of oncogene-dependent tumour biology and the success of driver mutation targeted therapy, all Stage IV lung cancers will eventually progress. Understanding the role of immunosurveillance in controlling tumour progression has been fundamental in the development of new immune based strategies for the treatment of lung cancer.43
The immune destruction of tumour cells is mediated by cross-talk between the adaptive and innate immune systems, and the tumour cells.44 Tumour cell elimination occurs when antigen-presenting cells recognise neo-antigens expressed on tumour cells and subsequently present them to T cells, priming these cells to affect an antitumour response. The ability of the tumour cell to escape immunosurveillance depends on the production of immunosuppressive cytokines; loss of major histocompatibility complex antigen expression; T cell inhibitory signals including increased expression of CTLA-4, PD-1, and its ligand PD-L1; and increased regulatory T (Treg) cells in the tumour microenvironment.45 Overexpression of PD-L1 in NSCLC, for example, inhibits primed T-cell activation and promotes immune evasion of the tumour.46
Immunotherapy takes advantage of these tumour features and has been a greatly successful strategy in lung cancer. The PD-1/PD-L1 pathway can be specifically targeted with a class of drugs known as immune checkpoint inhibitors. These agents have been trialled in first-line, second-line, and adjuvant settings in both early and late-stage disease, and across all NSCLC histologic subtypes. In the wake of a growing body of evidence, monoclonal anti-PD1 antibodies nivolumab and pembrolizumab, and the anti PD-L1 antibody atezolizumab, have firmly established roles in the treatment of advanced NSCLC. Landmark studies CheckMate-017 and CheckMate-057 used second-line nivolumab in patients with metastatic squamous and non-squamous NSCLC, respectively.47,48 Both studies showed improved overall survival and response rate, and reduced toxicity compared to docetaxel.49 Similar findings have been demonstrated for pembrolizumab and atezolizumab, and all three agents are now approved for second-line therapy in advanced NSCLC. At present, pembrolizumab is the only approved first-line single-agent treatment for advanced-stage NSCLC in tumours with a PD-L1 expression of ≥50%, with impressive improvements in overall survival when compared to standard platinum doublet therapy.50 In patients with unresectable Stage II and III disease, PD-L1 inhibitor durvalumab has also been shown to confer survival benefit when given as adjuvant therapy.51
Importantly, patients harbouring an EGFR mutation have not benefited from immune checkpoint inhibitors in these studies, possibly because tumours with a known driver-mutation characteristically have a reduced tumour mutational burden (TMB).49 Indeed, TMB has emerged as a promising biomarker for predicting treatment response. In the CheckMate-227 trial, combination therapy with nivolumab and ipilumimab (an anti-CTLA-4 antibody) demonstrated efficacy in comparison to standard first line platinum doublet chemotherapy in patients with a high TMB, irrespective of PD-L1 expression.52 Notably, increased immune-related adverse effects were observed in the combination therapy arm.
Although used in some studies for inclusion purposes, PD-L1 expression may not be the best biomarker for all check-point inhibitors. For instance, nivolumab and atezolizumab demonstrated efficacy in comparison to docetaxel in the second-line treatment setting, irrespective of PD-L1 expression.47,48,53 Furthermore, pembrolizumab in combination with platinum doublet chemotherapy, irrespective of PD-L1 expression, showed improvement in overall 1-year survival in patients with both squamous and non-squamous histology.54,55
Immunotherapy in Small Cell Lung Cancer
Despite the significant advances in precise and targeted treatment for NSCLC, therapy for small cell lung cancer (SCLC) has developed more slowly. The majority of SCLC is extensive-stage at the time of diagnosis, with median overall survival 8–13 months.56 Standard first-line therapy includes combination platinum and etoposide chemotherapy. Supporting the use of immune therapies in SCLC is their high immunogenicity, with an increased prevalence of associated paraneoplastic disorders. The IMpower133 trial has been practice changing, showing that the addition of atezolizumab to carboplatin and etoposide in previously untreated patients with metastatic SCLC led to clinically significant improvements in overall survival. Furthermore, this treatment effect occurred irrespective of the TMB.57 Studies of nivolumab, ipilimumab, pembrolizumab, durvalumab, and other immune checkpoint inhibitors in SCLC have also been conducted, with varying benefit.
Novel Approaches to Immunotherapy
Aside from targeting the PD-1/PD-L1 pathway, the immune response can be harnessed in other ways to affect an antitumour response. Two approaches under investigation include development of tumour specific vaccines, and manipulation of T-cells ex vivo to specifically target tumour cells. Overall, studies in lung cancer vaccines have been disappointing compared to those in immune checkpoint inhibitor therapy, perhaps due to the immunosuppressive tumour microenvironment.58 One strategy that has led to vaccine approval in Cuba is CIMAvax-EGF, a vaccine combining EGF with p64, which is a protein conjugate designed to enhance immunogenicity. In a Phase III randomised control trial of 405 Stage IIIB/IV NSCLC patients, CIMAvax-EGF vaccination resulted in a per-protocol median overall survival benefit, compared to best supportive care (12.43 versus 9.43 months, p=0.036).59
Adoptive cell therapy utilises T lymphocytes that have been isolated from the patient and genetically transformed to express a chimeric antigen receptor (CAR) targeted against a tumour derived antigen. CAR T-cells have mostly been studied in the setting of CD19 expressing haematological malignancies, with complete remission achieved in 68–100% of acute lymphocytic leukaemia patients.60 One of the primary obstacles for CAR T-cell therapy in solid organ cancers is identifying tumour antigens that are not also expressed by healthy tissue. It is possible that the antitumour activity of CAR-T cells may be optimised with the addition of immune checkpoint inhibitors, with clinical trials currently underway.61 A novel approach utilises CRISPR gene editing technology to destroy the PD-1 receptor, removing the inhibitory signal and thereby augmenting CAR-T cell cytotoxic tumour activity.62
FUTURE DIRECTIONS FOR PRECISION MEDICINE IN LUNG CANCER: BIOMARKERS AND TECHNOLOGY
The Role of Liquid Biopsies
Liquid biopsy is an emerging technology at the forefront of precision medicine in lung cancer, with potential for screening, diagnosis, and prediction of treatment response. It is a noninvasive method that can detect exosomes, circulating cell-free tumour DNA (cfDNA), cell-free tumour RNA (cfRNA), and circulating tumour cells (CTC).63 Liquid biopsies show great promise for cancer screening, however due to the rarity of CTC and tumour DNA products, extremely sensitive methods are required for their detection. Blood-based assays for detecting cfDNA, a chromatin DNA fragment, include PCR, droplet digital PCR, beads, emulsions, amplification and magnetics (BEAMing), and next-generation sequencing. A study using the sensitive method Cancer Personalized Profiling by deep Sequencing (CAPP-Seq) showed that cfDNA was detectable in 100% of Stage II–IV NSCLC patients, and in 50% of patients with Stage I disease.64 Interestingly in this study, cfDNA levels correlated with tumour volume. Due to a short half-life in circulation and potential for contamination with wild-type DNA, tumour-specific DNA can be difficult to isolate. Evolving technologies provide hope for future clinical application of cfDNA for diagnostic purposes.
CTC originating from tumour tissue can be detected with multiple techniques of varying sensitivities and specificities. A recent study demonstrated the presence of CTC in patients without evidence of clinically detectable lung cancer.65 The study included patients at risk for lung cancer, specifically those with chronic obstructive pulmonary disease. At baseline, 3% of the cohort had detectable CTC at baseline. Subsequent annual surveillance CT scans revealed the development of lung nodules 1–4 years after CTC detection. Early stage lung cancers were confirmed with resection. This study supports the fact that CTC migrate into the blood stream at an early stage of cancer development, potentially serving as a screening tool in high risk populations.65 As CTC can be morphologically heterogeneous, refinement of highly sensitive techniques for isolation has been challenging. Lack of standardisation of these methods has also limited their implementation into clinical practice to date.
Liquid biopsy specimens taken before, during, and after treatment can also elucidate tumour genomic changes over the course of the disease. In particular, this technology is clinically useful for the detection of drug resistance-related gene mutations.66 The use of cfDNA genotyping for detection of T790M mutations conferring resistance to EGFR-TKI treatment is now U.S. Food and Drug Administration (FDA)-approved.67
Lipidomics
In addition to liquid biopsies, the emerging science of lipidomics may also play a role in the early detection of cancer. Lipidomics, a branch of metabolomics, refers to the quantification of all lipids within a biological system.68 Lipids assist in membrane structure, storage of energy, and signal transduction in human cells, properties also utilised by cancer cells. Lipid metabolic profiles of serum from patients with early-stage NSCLC have been shown to be distinguishable from healthy controls and benign lung disease, showing promise as a biomarker for lung cancer diagnosis.69
Breathprinting
Analysis of exhaled volatile organic compounds can be undertaken to detect a cancer-related fingerprint, or ‘breathprint’, by an electronic nose. This approach to cancer diagnosis was derived from studies in trained household dogs, demonstrating the ability to distinguish exhaled breath samples of patients with lung and breast cancer from healthy controls.70 Subsequent studies of different electronic nose platforms for the early detection of lung cancer have shown high sensitivity (73–93%) and specificity (73–100%).71 Furthermore, there may be a unique volatile organic compound breathprint produced by tumours with EGFR, ALK, and p53 rearrangements, helping to facilitate noninvasive diagnosis and genotyping.72 Application of this technology is still translational and requires further validation prior to broader clinical use.
Radiomics and Deep Learning Techniques
Computational methods including radiomics and deep learning algorithms are developing technologies that can extract qualitative and quantitative data from radiological images, aiming to provide noninvasive biomarkers to aid with personalised clinical decision making. Radiomics refers to the quantification of radiological image texture, with subsequent correlation to clinical and genetic features, allowing a deeper processing of the image beyond the resolution of the human eye.73 A study utilising radiomics in a subset of the National Lung Cancer Screening Trial (NLST) data demonstrated high accuracy for predicting malignancy in nodules found on low dose CT.74,75 False positive rates were also much lower than in the original study (9.0% versus 96.4%). CT radiomic features (including air-bronchograms, ground glass components, pleural retraction, and tumour size) have also been correlated with mutations in EGFR, KRAS, and ALK genes in NSCLC.76-78 This combination of highly detailed radiologic and genomic data, so-called ‘radiogenomics’, has the potential to provide precise cancer characterisation as sophisticated technologies become increasingly available.
Deep learning algorithms are artificial neural networks that can be taught to recognise and interpret radiological patterns for diagnostic, therapeutic, and prognostic outputs. As with radiomics, deep learning has also been applied to the NLST low-dose CT data set, demonstrating superiority over human experts for predicting development of lung cancer, with AUC 0.94 and false positive rate 11%.79 The prognostic value of this technology was shown in a cohort of patients with surgical resection of NSCLC.80 In this study, deep learning networks outperformed traditional prognostic models such as tumour volume and TNM staging, with an AUC of 0.71.
Clinical Decision Support Systems
Clinical Decision Support Systems (CDSS) are electronic systems developed for clinicians, integrating a vast array of clinical data extracted from the electronic health record to inform management and surveillance decisions in cancer patients. Although currently limited in utility, CDSS for lung cancer patients have been shown to positively influence cancer care, and may foreseeably augment decisions made by the multidisciplinary team.81 Future CDSS that will incorporate radiomic and genomic data with other important clinical variables to enable therapeutic decision-making will offer tremendous advantages in the field of personalised medicine.
Summary of ‘Omics’ Data
Omics data, including the technologies described above, can be integrated to comprehensively understand the processes involved with cancer cell biology. There is huge potential for genomic and transcriptomic information to enable complex molecular profiling of individual cancers, giving new insights into tumour survival mechanisms, progression, and metastatic potential.82
CONCLUSION
The diagnosis and management of lung cancer have come a long way in the last decade, with increasing focus on a personalised approach. Novel technologies, such as liquid biopsy, omics data, and artificial intelligence present exciting opportunities for minimally invasive cancer diagnosis and characterisation. It is feasible that such techniques will serve as potential screening tools for populations at risk of developing lung cancer. The discoveries of driver mutations and key immunological pathways in many lung cancers have revolutionised therapy, highlighting the importance of accurate tumour characterisation. The development of resistance to targeted treatments continues to pose significant therapeutic challenges, requiring further interrogation into the underlying molecular processes promoting tumour growth. Further work needs to be done to elucidate patients who will derive the greatest benefit from available therapies, and to uncover tumour-specific biological processes that may be exploited for therapeutic purposes.