Abstract
Radical prostatectomy is widely used as the primary modality of treatment for clinically localised prostate cancer. A considerable proportion of men will have adverse histopathologic features and could benefit from adjunctive treatments: mainly adjuvant or salvage radiation.
This review focusses on the still unanswered questions:
- How to manage the patient after radical prostatectomy? Which patients need further treatment and which ones will not progress if not treated?
- How to refine patients selection for further treatments? What is the role for clinical nomograms and biomarkers and which ones are validated?
- When is it wise to propose adjuvant radiation therapy (RT) instead of observation with or without salvage RT?
- What is the optimal timing for the use of salvage RT and how to choose patients for each approach?
This article discusses the evidence available in the current literature, providing a critical analysis of the controversies of each strategy.
WHAT IS THE PROBLEM?
Radical prostatectomy (RP) with pelvic lymph node dissection and external beam radiotherapy are currently considered the standard treatment options for the management of localised, intermediate, and high-risk prostate cancer (pCa).1,2 In men treated with RP, the adverse histopathologic features, such as seminal vesicle invasion, positive surgical margins, and extraprostatic extension, are known to have a higher risk of developing biochemical recurrence (BCR), local recurrence, and clinical progression. For patients with noted adverse histopathologic features, the American Society for Radiation Oncology (ASTRO)3 and American Society of Clinical Oncology (ASCO) guidelines suggest considering adjuvant radiotherapy (ART), which could reduce BCR, local recurrence, and clinical progression risk. On the contrary, there is conflicting evidence about improvements in metastasis-free survival and overall survival.4-6
The European Association of Urology (EAU) guidelines1 clearly list two different options: immediate ART after recovery of urinary function or initial observation followed by salvage radiotherapy (SRT) in the case of BCR; the National Comprehensive Cancer Network (NCCN) guidelines2 provide similar recommendations, but also note that observation may be appropriate after RP.
ADJUVANT RADIATION THERAPY, SALVAGE RADIATION THERAPY, AND EARLY SALVAGE RADIATION THERAPY: DEFINITIONS
ART and SRT are the main modalities for radiotherapy (RT) administration after RP. Recently, the use of early salvage radiation therapy (eSRT) post-RP has been introduced. The main key differences between ART and SRT are the timing and oncological intent, while there is no significant difference in the radiation dose and irradiated field.
Currently, ART is intended for use in patients with undetectable prostate-specific antigen (PSA) after surgery, but the timing is still debated. Some urologists define ART as RT administered within the first 3 months after RP, while others wait until full recovery of urinary function, provided that PSA is still undetectable. SRT is used in patients with evidence of BCR or local recurrence, in the absence of distant metastases. BCR is currently defined as a post-RP PSA >0.2 ng/mL, although some authors have proposed higher cut-off values (PSA >0.4 ng/mL).7
eSRT is intended as administration of SRT at very low levels of PSA (from 0.03–0.20 ng/mL to 0.50 ng/mL),8 albeit the exact meaning and the biological significance of ‘very low PSA’ remains unclear and lacks consensus.9 A more definitive answer to this controversy will come from the ongoing randomised studies.
ADJUVANT VERSUS SALVAGE THERAPY: WHAT IS THE EVIDENCE?
Data from both the USA National Cancer Database (NCDB)10 and from a recent web-based survey among European urologists11 show that ART is underutilised worldwide. Hesitancy in offering routine ART may potentially be justified by evidence seen in randomised clinical trials comparing ART with initial observation;4-6 even if ART-treated patients had higher progression-free survival in comparison to observation, half of observed men were still free of BCR at 5 years (i.e. potentially a 50% overtreatment rate) and around one-third were free of BCR at 10 years. In these trials, there was a lack of overall and cause-specific survival improvement, with the exception of the SWOG8794 trial.4 Thus, not offering ART could reduce the risk of overtreatment, short and long-term side effects of radiation (up to 35% and 8%, respectively),4,12,13 which affect the genitourinary and gastrointestinal systems, and treatment expenses. However, besides the ARO trial, it might be argued that, in the SWOG and the EORTC trials, a post-surgical positive PSA was relatively frequent reflecting a dubious ‘adjuvant’ RT.
Notably, today the relevant questions lie in whether ART is superior to observation and eSRT in terms of cancer-specific and overall survival, and how might clinicians select between these approaches? The aforementioned trials did not provide a clear answer to this question, because SRT was not administered in the early setting as it is currently used. A retrospective study of 764 pT3pN0 patients with undetectable PSA after RP showed non-inferiority of ART to eSRT at long-term follow-up (>7 years) in terms of metastasis-free survival and overall survival.14 Additionally the results from three currently ongoing prospective multicentre open-label trials (RADICALS, RAVES, and GETUG-17) are pending.
Current evidence for eSRT is mostly based on retrospective studies, with BCR as the primary outcome and without considering clinical progression, cancer-specific survival, and overall survival. Pfister et al.15 reviewed 10 retrospective studies related to eSRT, and concluded that eSRT had good biochemical relapse-free survival of 71% (48.0–81.8%) at 5-year follow-up. Of note, the authors discovered an almost linear association of lower PSA values at eSRT and improved biochemical relapse-free survival, with an optimal PSA threshold of roughly 0.50 ng/mL (or even lower, according to Siegmann et al.16). Unfortunately, no direct comparison between ART and SRT was made. These results were confirmed by Stish et al.,17 who also reported a strong association between lower PSA level and distant metastasis, cancer-specific death, and overall survival.
Briganti et al.18 investigated a large multi- institutional cohort of 890 pT3pN0, R0/1 pCa men who underwent ART or observation with or without eSRT (PSA <0.50 ng/mL). With regard to recurrence-free survival, the results demonstrated the non-inferiority of ART to eSRT at 2-year and 5-year follow-up. Fossati et al.14 confirmed comparable effectiveness between ART and eSRT in terms of overall survival and metastasis-free survival in a similar multi-institutional cohort of 510 patients at almost 8-years follow-up.
THE DECISION-MAKING AFTER RADICAL PROSTATECTOMY: CONVENTIONAL TOOLS
The first instrument that was predictive of BCR after RP, brachytherapy, or external radiotherapy, was developed by D’Amico et al.19 in 1998, stratifying men on the basis of pretreatment PSA level, biopsy Gleason score, and clinical T stage. Additionally, a study performed at the Mayo Clinic found that this D’Amico stratification was able to predict not only BCR but also clinical progression and survival.20 A different way of addressing the problem is to summarise the most important clinical variables into a scoring system. The most relevant of these are the Cancer of the Prostate Risk Assessment post-surgical score (CAPRA-S),21 the Stephenson postoperative nomogram,22 and the new postoperative nomogram proposed by Dell’Oglio et al.23 specifically aimed at men already experiencing BCR. Although these clinically based nomograms (Table 1) are often used in decision-making for patients after surgery, none of them have specifically been validated for identifying which patients will benefit from ART rather than observation with or without SRT.

Table 1: Summary of described nomograms on predictive accuracy and reported outcomes.
ADT: androgen deprivation therapy; BCF: biochemical failure; BCR: biochemical recurrence; CI: confidence interval; CSM: cancer-specific mortality; pCa: prostate cancer; PSADT: prostate-specific antigen doubling time; RP: radical prostatectomy; SRT: salvage radiation therapy.
Abdollah et al.24 developed a risk score based on several histopathological adverse features (positive margins, extracapsular extension, seminal vesicle invasion, pT4 stage, nodal invasion) in patients who underwent RP and extended lymph node dissection. Despite several limitations (inclusion of node positive patients [37.7%]; unstandardised use of both ART and hormonal therapy [41.6% of patients, with an uneven distribution between the ART and non-ART groups]; non-separation of pT3b from T4), the study provided some valuable insights. Most notably, ART significantly improved overall survival and cancer-specific survival only in patients with at least two risk factors out of pGS ≥8, Stage pT3b/4, and positive lymph node count >1.
With regard to SRT, Tendulkar et al.25 recently updated a nomogram based on concurrent androgen-deprivation therapy, pathological Gleason score, extraprostatic extension, surgical margins, seminal vesicle invasion, pre-SRT PSA, and SRT dose. Not surprisingly, a collateral finding was that early SRT (for PSA level <0.50 ng/dL) after RP led to improved BCR and distant metastases survival.
Furthermore, recent studies found that the development of BCR was not sufficient to suggest patients should receive SRT, as roughly one-third (22.9–37.0%) developed clinically evident recurrence,26,27 and only 1 out of 20 died from pCa (5.8% of a cohort of mostly low and intermediate-risk pCa patients). Using Brockman et al.’s nomogram28 on the basis of pathological features from prostatectomy specimens (pathological Gleason score, extraprostatic extension, seminal vesicle invasion), time to BCR, preoperative PSA, PSA level at BCR, and PSA doubling time (PSADT; the last one is not strictly necessary), authors developed a predictive tool (area under the receiver operating curve [AUC]: 0.763) for pCa mortality in men with BCR. Of note, however, is that while Brockman et al.’s nomogram accuracy does not rely heavily on PSA doubling time (AUC: 0.754 without PSADT), several authors proved it to be a relevant prognostic factor for systemic progression29,30 and cancer-specific mortality31 in patients with BCR. There is a general agreement for administering SRT for a PSA doubling time shorter than 6 months32 and to defer any therapy in men with a PSA doubling time longer than 12 months.33
Currently, standard SRT is considered for a PSA value >0.50 ng/mL, but over the past several years there has been interest in lower PSA threshold for SRT administration (early SRT: radiation therapy administered for 0.20 ng/mL<PSA<0.50 ng/mL; or ultra-early salvage radiation therapy, ueSRT: radiation therapy administered for 0.01 ng/mL<PSA<0.20 ng/mL).
Among the most interesting studies, Fossati et al.34 investigated 716 pN0 patients with undetectable postoperative PSA who underwent eSRT for PSA recurrence (i.e. two or more 0 ng/mL<PSA≤0.50 ng/mL). The authors found that each 0.1 ng/mL PSA increase was associated with an overall 3% risk increase of BCR at 5 years; patients with at least two risk factors (including pT3b/pT4 disease, pathologic Gleason score ≥8, and negative surgical margins) have a 10% risk increase of 5-year BCR for each additional 0.1 ng/mL PSA increase, while men with one or no risk factors have a 1.5% risk increase of 5-year BCR for each additional 0.1 ng/mL PSA increase. The researchers concluded that administering eSRT at the first sign of PSA rise (>0.1 ng/mL), without waiting for the 0.50 ng/mL conventional threshold defining BCR, could confer better cancer control in high-risk patients. The available evidence on very early SRT remains controversial, with studies both in support35 and against.36
There is no clear role for imaging in eSRT patient selection; current EAU guidelines themselves1 do not recommend eSRT unless there are specific concerns about systemic spread. Data using endorectal-coil magnetic resonance imaging (MRI) with contrast enhancement are contrasting; Linder et al.37 reported an overall sensitivity of 91% and a specificity of 45% (median post-RP PSA 0.59 ng/mL) with a sensitivity of 86% in patients with PSA <0.4 ng/mL. On the contrary, Liauw et al.38 reported a sensitivity of only 13% with PSA level <0.3 ng/mL. Thus, clear-cut application in everyday clinical practice is currently not feasible. A review by Vees et al.39 underlined a comparable scenario for 18F-choline and 11C-acetate positron emission tomography–computed tomography (PET/CT) in the post-RP early BCR setting (55% of studies in support and 45% studies against). More promising outcomes have recently been seen with the introduction of Ga-prostate-specific membrane antigen (PSMA) PET/CT, which has proved efficacious in the evaluation of men with BCR and 0.05 <PSA<1.0 ng/mL40,41 with a roughly 50% detection rate for PSA <0.50 ng/mL, and 28.6% detection rate for 0.20 ng/mL<PSA<1 ng/mL in 8F-choline negative patients.42 Valuably, a refined imaging strategy may not only help to properly confirm or exclude a local recurrence but also improve its management (e.g. deliver a boost dose with RT to the suspected macroscopic relapses).
BIOMARKERS: NEWER TOOLS TO GUIDE DECISION-MAKING AFTER RADICAL PROSTATECTOMY
In the last 10 years, numerous genomic and proteomic tests have been developed in order to refine patient selection among patients with newly diagnosed pCa, post-prostatectomy patients, systemic-disseminated pCa, and hormone-refractory pCa patients, and both improve outcomes and tailor indication for possible further therapies. Genomic predictive tools look very promising in the field of outcome prediction after RP, especially when integrated into a clinical model.43,44 Ascertaining the most reliable predictive tools remains a challenge. A large number of gene mutations and genomic alterations are involved in prostate tumour carcinogenesis. The aforementioned features are the basis used to develop stand-alone genomic classifier predictive scores, or to empower a pre-existent clinical one, and the information provides an estimate of risk of metastases after RP.45,46 The most promising biomarkers are discussed below and summarised in Table 2.
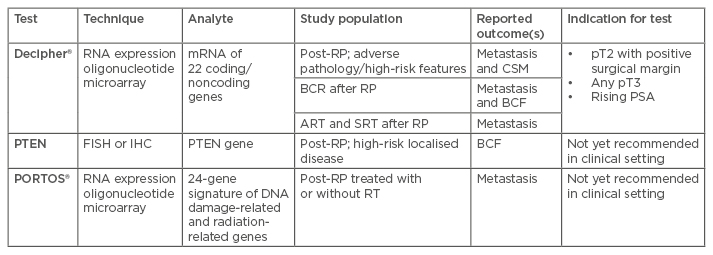
Table 2: Summary of genomic assays available for post-surgical setting.
ART: adjuvant radiation therapy; BCF: biochemical failure; BCR: biochemical recurrence; CSM: cancer-specific mortality; FISH: fluorescent in situ hybridisation; IHC: immunohistochemistry; PSA: prostate-specific antigen; RT: radiation therapy; RP: radical prostatectomy; SRT: salvage radiation therapy.
Decipher®
Decipher® is a 22-RNA biomarker panel genomic classifier tool developed by GenomeDx Biosciences (Vancouver, Canada) to predict post-RP pCa outcomes. It is based on differential gene expression and transcription in non-recurrent and recurrent pCa. Tissue specimens from RP are processed to extract RNA and analyse over 1.4 million coding and non-coding genomic regions. Absence/presence of 22 selected markers involved in cell differentiation, proliferation, adhesion, motility, structure, cell-cycle progression, mitosis, immune modulation, and other unknown functions are used to generate a score (ranging from 0–1), known as the Genomic Classifier (GC) score.47 The GC score can predict BCR, metastasis, and pCa-specific mortality after RP.
The GC score has also been externally validated; Ross48 and Karnes49 confirmed that GC score predicts metastatic progression with good accuracy. Den et al.50 tested GC score in the setting of post-RP radiotherapy (both ART and SRT). One hundred and eighty-eight post-RP and post-RT (in an ART or SRT setting) patients were retrospectively studied with regard to the risk of metastatic progression. Although bias was present (retrospective analysis, equivocal selection criteria for ART versus SRT, non-standardised use of ADT), GC outperformed CAPRA-S in outcome prediction; A GC score >0.6 seemed to identify a cohort of high-risk patients who most benefitted from ART (80% hazard reduction in Cox models).
Den et al.43 evaluated GC score in a cohort of 139 patients with adverse features at RP (i.e. pT3 or positive surgical margins) compared to a clinical score based on the Stephenson nomogram, finding them equivalent in predicting BCR and metastases-free survival (AUC of 78% and 80%, respectively). These studies suggest that patients with higher GC scores could benefit from ART, even if their risk of BCR and metastatic progression is higher, while patients with lower GC scores could most benefit from observation and eSRT. The same results were achieved by Den et al.51 investigating outcomes from 422 post-RP men with pT3 disease or positive margins.
Freedland et al.44 analysed the predictive value of GC score for metastases risk in post-RP and SRT patients, finding that GC score performed better than the Briganti and CAPRA-S nomograms (GC AUC: 0.85 versus Briganti nomogram AUC: 0.65 and CAPRA-S nomogram AUC: 0.63). Klein et al.52 applied GC scores to low-risk pCa (i.e., Gleason score: 6) confirmed at RP, and found higher GC scores (>0.60) in 7% of patients despite low-risk disease.
Decipher was confirmed to be a strong predictor of metastases risk progression even when applied to prostate biopsy specimens,53 especially when used in conjunction with NCCN risk groups (C-index for NCCN alone: 0.75; for combination NCCN with Decipher: 0.88). This amount of evidence drove the NCCN guidelines to recommend Decipher to predict metastases risk in post-RP patients with adverse features (i.e. pT3 or positive surgical margins) or BCR.2 Despite valuable and promising insights, Decipher use to predict metastases risk after SRT still requires further validation.
Phosphatase and Tensin Homolog
The phosphatase and tensin homolog (PTEN) gene is a tumour suppressor gene involved in the PI3K/AKT signalling pathway, which promotes cell survival and proliferation. Several studies identify PTEN as one of the most commonly mutated or deleted tumour suppressor genes in pCa (10–70% of pCa show PTEN loss).54 PTEN deletion is predictive of shorter recurrence-free survival55 and higher risk of cancer-related death.56 Cancer-related death risk has been found to be higher when PTEN and TMPRSS2-ERG gene deletions are associated.57 At the moment, clinical application of PTEN still lacks strong external validation and further evidence is required for recommendation in guidelines as a standardised biomarker.
Post-Operative Radiation Therapy Outcomes Score
Post-Operative Radiation Therapy Outcomes Score (PORTOS) is a biomarker proteomic tool based on protein expression of a panel of 24 genes involved in DNA damage response or in immune response, which predicts individual response to RT after RP. PORTOS provides a number between 0 and 1; a lower PORTOS score identifies patients who benefit less from radiation, while a higher PORTOS score identifies men most likely to benefit from RT. This feature makes PORTOS the first validated genomic test that might predict pCa response to postoperative radiotherapy, even if further validation is needed before applying in everyday clinical practice. Zhao et al.46 confirmed the capacity of PORTOS to refine patient selection for RT. In a cohort of 196 patients, those with a higher PORTOS treated with RT experienced a significantly lower rate of metastatic progression when compared with patients who had comparable PORTOS but did not receive radiation after RP (5% and 63%, respectively, at 10-year follow-up; hazard ratio: 0.12; p<0.0001). Furthermore, authors reported that Decipher, the microarray version of Prolaris® (mCCP), and CAPRA-S were not able to predict the response to postoperative radiotherapy, while PORTOS does.
FUTURE SCENARIOS IN BIOMARKERS:ONGOING RESEARCH AND DEVELOPING TOOLS
Current research about predictive tools can be divided into several categories: studies addressing patients with newly diagnosed pCa, studies addressing decision-making after RP, and those to improve management of systemic-disseminated pCa and hormone-refractory disease. As for tools, we are receiving a flourishing amount of research for genomic and proteomic biomarkers, in order to develop so-called genomic and proteomic tools, both stand-alone and nested in a pre-existent clinical tool. Nevertheless, even if knowledge about pCa biology, development, and progression pathways has increased in the past few years, we still need further information to fully understand this disease and to personalise therapies for each patient. Data from the Catalogue of Somatic Mutations in Cancer (COSMIC) database show that pCa is the expression of about 80,000 DNA mutations of a discrete number of genes: most frequently, TP53 (14%), AR (8%), SPOP (8%), PTEN (7%), KMT2C (5%), FOXA1 (5%), KRAS (4%), and KMT2D (4%). These genes, which are usually involved in cell proliferation and survival, are thought to co-operate in pCa development, and the fact that none of them show a strong prognostic and/or predictive value in oncological outcome on its own seems to confirm this hypothesis. Thus, developing a comprehensive, affordable, but effective biomarker tool remains a challenging effort.
FUTURE PERSPECTIVES
Prospective trials allow researchers to develop a unique study protocol but may take time to develop, have long recruitment times, and the outcomes could be outdated as soon as they are ready to be published. On the other hand, retrospective studies give scientists less control over patient follow-up and treatment but outcomes can be analysed quickly, despite the potential for bias. For this reason, we believe that good-quality retrospective evidence and large multi-institutional prospective studies both have a relevant role in providing answers to the aforementioned questions, and many others to come. The trend towards a patient-tailored therapy and the idea of a potential multi-modal pCa treatment seem to be fundamental; improving the knowledge of pCa biology seems to be a major way to assess individual risk and personalise therapies.