Abstract
The integrative action of the autonomic nervous system (ANS) and its cellular constituents promotes the harmonic cooperation between different organs and functional units of the body. Research into its structure and physiology has promoted key advances in system, cellular, developmental, and clinical neurosciences and brought forward a range of milestone concepts central to neuroscience.
ANS dysfunction in multiple sclerosis entails a range of prominent challenges concerning cardiovascular control, thermoregulation, and pelvic organ function. They not only derail the smooth interaction of bodily functions but also negatively impact the course of the disease. With the resolution of central questions on ANS function, different strategies and pharmaceutical approaches emerged to handle these autonomic dysfunctions.
INTRODUCTION
Multiple sclerosis (MS) is first perceived as a disease affecting voluntary motor and sensory systems. The autonomic dysfunctions associated with the disease receive less publicity yet pose a major burden because they critically affect the daily lives of patients and deteriorate the course of the disease. Because of the tight interplay between the study of the autonomic nervous system (ANS), the development of major concepts in neuroscience, and the progress in drug development, their comparative discussion makes a showcase for the progress and the shortcomings of battling such a complex disease.
A Short History of the Autonomic Nervous System
With the monographs of John Newport Langley (1921)1 and Walter Holbrook Gaskell (1920),2 the autonomic or involuntary nervous system became a well delineated target of exploration in a range of biomedical sciences. They addressed the questions of how this system operates, how it develops, and how it is altered in disease.
Physiological and pharmacological studies throughout the 20th century shaped the picture of the ANS as a key to the neurobiology of homeostasis.3 In addition, developmental studies promoted insight into the generation and integration of its cellular elements into autonomic neural circuits.4 Moreover, molecular analysis reshaped current understanding of the distinct ANS domains into developmentally and molecularly distinct branches.5
Already in the 18th century, Jaques–Benigne Winslow (1732)6 attributed the sympathies of the body to a set of nerves, including the great sympathetic, then called the intercostal nerve, and the medium sympathetic nerve, the par vagum. The subdivision of the ANS into sympathetic and parasympathetic domains was then established on anatomical, physiological, and pharmacological grounds by Langley1 and Gaskell.2 The demonstration of the interaction in the regulation of the heart and other organs resulted in a picture of two largely antagonistic nerve nets whose integrative power adjusts the organs performance to the actual bodily requirements.
The cardiovascular control in fact constitutes one essential task of the ANS appropriating the local blood supply while synchronising blood flow and temperature control. The ground-breaking work by Otto Loewi and Henry Hallett Dale paved the way for understanding this communication between the nervous system and target organs such as the heart. In his landmark study on the action of the vagus and sympathetic nerves on frog hearts, Loewi in 19217 demonstrated the release of soluble substance(s) mediating the nervous information to the target organ. The Vagus Stoff, the mediator of vagus nerve stimulation that was postulated by Loewi, was characterised by Dale as acetylcholine released by the nerve.8 Only later, the active substance released by sympathetic nerves was identified as noradrenaline by von Euler.9,10 Consequently, the antagonistic interaction of the sympathetic and parasympathetic system (not only into the heart) became understood in an initial, oversimplified model as the balance between noradrenergic and cholinergic transmission to the target organs.
A Short Glimpse at the Autonomic Dysfunctions in Multiple Sclerosis
Since the publication by Wilhelm Uhthoff in 189011 on visual disturbances in patients with ‘multiple Herdsklerose’ as a consequence of physical exercise and increases in body temperature, heat intolerance and thermoregulatory dysfunction12,13 are recognised as key pathophysiological features in MS.
The delineation of the precise causes of heat intolerance poses a complex enterprise as disturbances in the peripheral sensory, central processing, and peripheral efferent pathways may be involved. Alterations in sweat production, one regulatory arm operated directly by the sympathetic nervous system (SNS), in individuals who are experiencing relapsing remitting MS14 point to a compromised integration of the SNS outflow as contributing to the compromised thermotolerance under load.15
In addition, orthostatic intolerance is frequently detected in MS patients16 by heart rate and blood pressure measurements, albeit cardiovascular autonomic dysfunctions figure less prominent than, for example, in Guillain-Barré disease. Yet, the lower than normal SNS activity17 is considered to contribute not only to cardiovascular problems but also to immune inflammatory and neurodegenerative processes, promote osteoporosis, and favour fatigue and depressive episodes.18,19 Thus, sympathetic dysfunction may not only be a consequence of the disease but also affect its course. While sympathetic dysfunction correlates with the clinical activity of MS, parasympathetic dysfunctions are indicators of the progression of disability.20
Despite the limitations posed by compromised cardiovascular and temperature regulation, dysfunctions of the pelvic organs may have more dramatic effects on the lives of individuals with MS. Disturbed control of bladder21-23 and bowel24,25 may not only have serious consequences on the daily lives but also result in secondary complications, in particular urinary tract infections. In addition, sexual dysfunction may be a feature in MS.26,27 The lumbar and sacral ANS is central to the co-ordination of these pelvic organs’ function. Developmental analysis of the sacral pre and post-ganglionic components demonstrated close relationship to the thoracolumbar domains and reclassification of this entire region as sympathetic.5
THE HISTORY OF SCIENTIFIC CONCEPTS ON AUTONOMIC NERVOUS SYSTEM FUNCTION AND THE ADVANCEMENT OF THE THERAPEUTIC OPTIONS FOR AUTONOMIC NEUROGENIC DYSFUNCATION
The Search for the Transmitting Principle
With the work of Langley1 at the turn to the 20th century, a decisive change in the experimental approaches to ANS function was introduced in the use of diverse purified natural substances such as adrenaline, nicotine, curare, or pilocarpine. One critical observation was that stimulation by injection of adrenaline in an experimental animal elicited effects similar to those of sympathetic nerve stimulation by brief tetanising inductive shocks, whereas application of pilocarpine provoked effects similar to stimulation of parasympathetic nerves. In addition, Langley observed that the ‘end apparatus’1 of the nerve fibre was responsible for paralysing effects of curare acting on preganglionic fibres to then raise the question for the ‘receptive substance’1 that mediates the action in the target tissue. Thus, Langley’s work opens the search for the transmitting principles of neurones which is to become one key topic in the neurosciences as well as a main road in drug development. This entails the question for the ‘receptive substance’ as well as the ‘end apparatus’.
Proof that the transmitting principle may be a soluble substance released from the nerve was provided by Loewi7 at the same time that Langley published his monograph. The identification of this substance by Dale8 as acetylcholine resolved the molecular identity of the first classical neurotransmitter molecule. In addition, Loewi provided evidence for the release of an adrenaline-like substance as a result of sympathetic nerve stimulation. Although the opinion that adrenaline is the sympathetic mediator held until the late 1930s, a range of discrepancies, in particular pharmacological in nature, ultimately culminated in the demonstration of noradrenaline as the sympathetic neurotransmitter.10
The Quest for the Receptive Substance
At the turn to the 20th century the structure of adrenaline, the active principle of the adrenal medulla, became uncovered due to the work of Abel, Takamine, Fuerth, Aldrich, and others (see references).28 At the same time, structurally related substances became available for physiological experimentation. The studies demonstrated that ‘an action simulating that of the true SNS is not peculiar to adrenine, but is possessed by a large series of amines’ and coined the term ‘sympathomimetic’.28 The comparison of the effects of these sympathomimetic substances on different cardiovascular parameters in a range of mammalian species provided evidence that there are ‘two types of adrenotropic receptors’.29 ‘The alpha[…]receptor is associated with most excitatory functions (vasoconstriction…) whereas the beta receptor is associated with most of the inhibitory functions (vasodilation…)’.29
Further characterisation, isolation, and cloning unfolded the diversity of the receptor family members involved in adrenergic signalling. With the purification and isolation, by affinity chromatography with conjugates, of noradrenaline linked to agarose beads from canine ventricular myocardium, the hypothetical ‘receptive substance’1 or ‘adrenotropic receptor’29 turned into a ‘physicochemical entity’, the β-adrenergic receptor.30,31 The molecular cloning of the receptors, starting with the mammalian β2 adrenergic receptor,32 turned into the identification and structural characterisation of the two families of α and β adrenoceptors33 underlying adrenergic and noradrenergic regulatory signalling. Also, the muscarinic receptors mediating acetylcholine signalling in autonomic target tissues constitute a gene family, and comparison with adrenoceptor genes demonstrated that the gene families are closely related.34
The Diversity of Transmitter Receptor Molecules – A Road to Improve Sympathomimetic Specificity
The observation of different types of adrenergic receptors in the diverse autonomic target tissues29 was complemented by the demonstration of tissue-specific expression patterns as described already for the first cloned receptor genes.32 The findings allowed plausible explanation of the wide ranging effects of sympathomimetic drugs characterised by a combination of desired therapeutic actions and a rich spectrum of side effects. Together, with the reports of different actions of distinct adrenaline-related amines on various target organs,28 they fed the vision that structural modification of sympathomimetics would allow the generation of more specific drugs. This is well illustrated with the development of sympathomimetic bronchodilators,35 starting from the ‘original autonomic drug’ adrenaline.36
With the development of salbutamol by the group of David Jack in 1968, a key drug in respiratory medicine became available37 that acts as specific β-2 adrenoceptor agonist. In addition to the effects of β adrenoceptor agonists on asthma and obstructive airway disorder, β-2 agonists are discussed as add-on therapy in MS. Apart from improving sympathovagal balance and cardiovascular dysfunction in MS, the use of sympathomimetics goes beyond treatment of these basic autonomic functions.38,39 The involvement of adrenoceptors in the neuro–immune connection and the role of β-2 adrenoceptors in inflammatory demyelination and axonal degeneration point out different alleys along which modulation of the ANS and/or adrenoceptor function may impact the course of the disease.
Interestingly, antagonists for muscarinic acetylcholine receptors were also used early on for purposes overlapping with the bronchodilatory effects of the adrenoceptor agonists. Due to the general spasmolytic effects, much effort has been devoted to the development of specific antimuscarinergic drugs targeting the individual members of the receptor family in different organs. In the case of asthma and obstructive pulmonary diseases, a set of substances is available with increased specificity for M3 muscarinic receptors40 that may be combined with adrenergic agonists. Most relevant for individuals with MS is the anticholinergic therapy for the treatment of lower urinary symptoms.23 In MS with diverse manifestations of the symptoms24 this figures prominently to treat overactive bladder syndromes.22 A number of different drugs are available with some preference for M3 receptors.
The Signal Transduction Mechanisms – the Advent of the Second Messengers
The purification and identification of the adrenoceptor as ‘physicochemical entity’31 merged with the discovery of cyclic adenosine monophosphate (cAMP) and characterisation of cAMP as ‘second messenger’.41 This fusion of knowledge between physiology and biochemistry had a major impact on drug development well into the 21st century.
The reconstitution of adenylate cyclase (AC) activation upon adrenoceptor activation in heterologous and depleted systems42 demonstrated that the signal transduction initiated by a neurotransmitter and its receptor could be deciphered at the level of single protein interactions. This observation paved the way to deconstruct the cellular signal transduction pathways down to intricate molecular details.
The picture diversified into different directions. In the 1980s, it was recognised that AC may be under dual control,43 paying tribute to the diversity of signalling molecules and their receptors, i.e., adrenergic and muscarinic. The characterisation of different GTP-binding proteins (G proteins) that provide the connection between receptor and AC explains part of the activating versus inhibiting mechanisms.44 The diversity of AC encoded by a gene family poses another level of complexity.45
Thus, in a long-standing effort it was shown that activation of AC by adrenoceptors constitutes the primary mechanism to increase cardiac performance in the heart under load,46 where the equilibrium between AC and phosphodiesterases (PDE) dictates cAMP/PKA activities.47,48
Roughly a decade after the characterisation of cAMP,49 cyclic guanosine monophosphate (cGMP), and a guanylate cyclase were described.50,51 Consequently, transduction of hormonal and neurotransmitter signalling in cells was recognised to rest on the cyclic nucleotides cAMP and cGMP. The regulation of their synthesising enzymes and the degrading PDE turned into a key focus of basic science and drug development. These scientific milestone discoveries were awarded the Nobel prizes in Physiology or Medicine for Earl Wilbur Sutherland in 1971, for Alfred Goodman Gilman in 1994, and in Chemistry for Robert Joseph Lefkowitz in 2012.
Additional Transmitting Substances
In addition to the two classical transmitters noradrenaline and acetylcholine, a range of neuromodulators have been described to fine-tune autonomic neurotransmission. Already in the 1970s, purines like ATP and peptides like neuropeptide Y, somatostatin, and vasoactive intestinal polypeptide were recognised to play important roles in autonomic neurotransmission.52,53 Importantly, receptors for the nonclassical neurotransmitters turned out to, at least in part, couple to G proteins.52
A most surprising member of the neuromodulator group, nitrogen monoxide (NO), a substance originally known as a caustic pollutant in the earth’s atmosphere, was characterised to exert dramatic effects on guanylate cyclase activity in target cells, in particular vascular smooth muscle.54 Starting with the observation that NO is able to activate the cytosolic form of guanylate cyclase,55 it could be shown that NO is able to relax vascular smooth muscle cells by affecting cGMP levels.56 In the following, the field of cardiovascular regulation by cyclic nucleotide second messengers turned into the most rapidly expanding field in biomedical sciences.54 The balancing of cGMP and cAMP and the role of their degrading enzymes, the cyclic nucleotide PDE, as therapeutic targets became a focus of interest.
With the emergence of inhibitors of the PDE, a quantum leap was achieved in the treatment of male erectile dysfunction (ED). This autonomic dysfunction also affects patients with MS.26,27 In an attempt to develop inhibitors against PDE5 for the treatment of hypertension and angina pectoris, a substance, discarded for its lack of the desired effects, attracted attention for a side effect. Sildenafil, a highly specific PDE5 inhibitor,57 revolutionised the treatment of ED by supporting the desired vasodilatory effect in the appropriate contextual setting and with high tolerability.58 With the excellent efficacy and tolerability profile, a series of drugs with comparable effects were rapidly developed. Among the autonomic dysfunctions, in particular of the pelvic organs, the success in the treatment of ED is outstanding.
The Search for the End Apparatus: A Highly Complex Target for Toxins
With the breakthroughs demonstrating how information propagation between neuron and target cell is mediated by soluble transmitter molecules and the understanding of the receptive process on the target cell being mediated by receptor proteins, the release of the transmitter from a neuron remained a key question in cellular and molecular neuroscience. Refined electrophysiological techniques opened the window into the nature and kinetics of the release process. Modern methods of protein purification in combination with molecular cloning, targeted inactivation, and expression techniques enabled biochemists to characterise the molecular players of the synapses at the nerve terminal.59
Various toxins such as the clostridial tetanus toxin and botulinum toxin (BoTX)60 supported the characterisation of individual proteins in the synaptic protein complexes. These toxins degrade specific proteins associated with presynaptic plasma membrane and synaptic vesicles required for calcium-induced fusion of the transmitter-containing vesicle and consequent neurotransmitter release. The molecular methods to express and/or manipulate individual synaptic proteins allowed to describe their specific functions61,62 and to understand the vesicle cycle as the interaction of a diverse set of synaptic proteins.
In a surprising conversion of toxic to beneficial function,63,64 controlled local application turned BoTX into a therapeutic tool. Taking advantage of the specific uptake of toxin subunits into nerve terminals and degradation of individual SNARE complex proteins critically involved in calcium-dependent synaptic vesicle fusion,65 BoTX can turn down the release of neurotransmitters. In this manner, activity of selected targets can be reduced or blocked by localised application of the toxin. For MS patients, overactivity of the detrusor muscle can be reduced in some manifestations of the neurogenic bladder.66 This allows an approach to the voiding function in cases where dysfunction is refractory to anticholinergic therapy or when its side effects prevail.
THE INTERDIGITATION OF AUTONOMIC NERVOUS SYSTEM AND IMMUNE SYSTEM FUNCTION AND THE IMPACT OF IMMUNE MODULATORY THERAPIES
An issue of particular relevance is the interaction of the ANS and the immune system and its disturbances in MS.19,67,68 This entails a range of different aspects concerning the functional interaction in the two systems where the sympathetic nerve plays a critical role as ‘integrative interface’.69 Here, the autonomic innervation of primary, secondary, and tertiary lymphoid organs is of essence.70,71 Unbalanced sympathetic activity in the spleen is discussed to drive immune-mediated inflammatory activity.72 Disease-mediated alterations in the properties of lymphocytes and associated cell types in lymphatic tissues include changes in adrenoreceptor expression on lymphocytes in MS.73-75 The combined effects of such processes at the meninges, a gathering place for diverse types of immune cells richly innervated by autonomic neurons,76 is still unresolved. In addition, challenges secondary to the immediate consequences of the dysfunction in nervous and immune system may be critical. Of particular interest are disturbances in the voiding functions, particularly lower urinary tract symptoms and associated bacterial infections.77 How significantly the alterations in gut motility and function imposed by autonomic dysregulation add to this complexity78 is a lasting question.
A critical issue in this context concerns the effect of immune modulatory therapies on the functions exerted by the ANS. Treatment of acute exacerbations in MS with high doses of methylprednisolone has the potential to induce cardiac arrhythmias and conduction disturbances.79 For immune modulatory approaches to manage long-term course and morbidity after MS diagnosis, the picture is as diverse as the arsenal of substances. The first significant step forward has been achieved with β interferon preparations which, during the initial years of searching, were praised for their absence of important side effects.80 Indeed, no differences were found in autonomic scores for cardiovascular functions in a placebo-controlled study.20
A different situation is found for the immune modulatory drugs mitoxantrone and fingolimod that may compromise cardiovascular function on different timescales.81 Via its action on sphingosine 1-phosphate receptors (S1PR), fingolimod may result in the desired action on lymphocyte sequestration via subtype 1 S1PR expressed in lymphocytes. In addition, transient, occasionally severe, cardiac side effects may be elicited via other receptor subtypes.82,83 Parasympathetic as well as sympathetic cardiac drive may be affected.84 Differently, long-term treatment with mitoxantrone, a DNA topoisomerase inhibitor, may lead to cardiomyopathy associated with mitochondrial dysfunction.85 As such, different immunotherapies acting via entirely distinct mechanisms may induce off-target effects on autonomic functions of very different type and scale.
CONCLUSIONS
Since the description of a conspicuous heat intolerance by Uhthoff in 189011 in patients with sclerotised lesions in central nervous system white matter, autonomic dysfunctions emerged as a frequently observed catalogue of symptoms severely impacting quality of life in people affected by MS. During the very same time at the end of the 19th century, Langley and Gaskell performed their ground-breaking anatomical, physiological, and pharmacological studies on the ANS that were summarised in their monographs on the ‘Autonomic Nervous System’ by Langley in 19211 and the ‘Involuntary Nervous System’ by Gaskell in 1920.2
At that time, the pharmacological approach used by Langley opened a series of key questions shaping the molecular neurosciences during the 20th century: the imminent problem of the information transmitting principle of autonomic neurones, the in vivo pendants to adrenaline and pilocarpin, the nature of the receptive substance, and the properties of the end apparatus responsible for communicating neural activity to the target organ. Each of these key problems yielded rich molecular knowledge on basic mechanisms in nervous system function resulting in a detailed picture of the working of cellular and molecular autonomic neuroscience (Table 1).
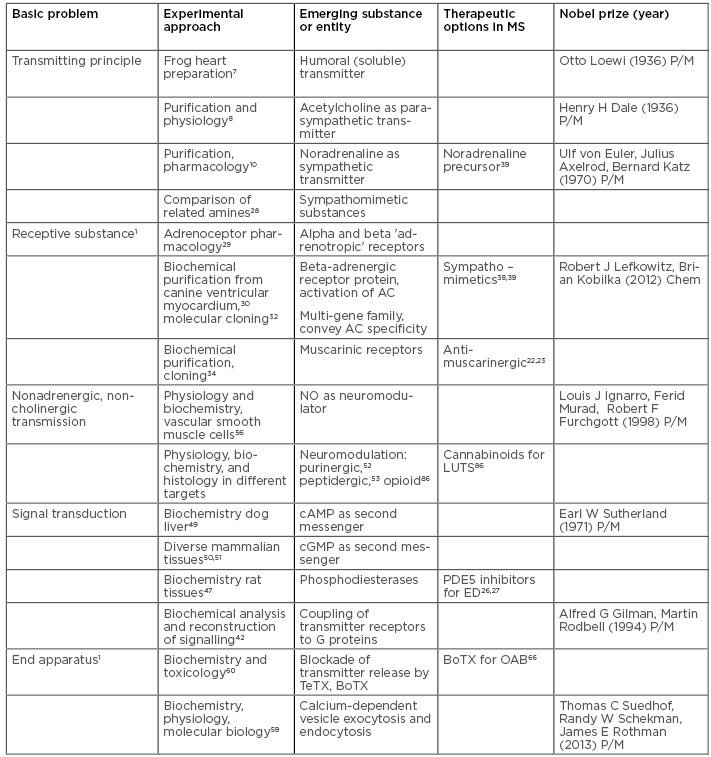
Table 1: The evolution of key concepts on autonomic neurotransmission and the development of drugs affecting these transmission pathways in multiple sclerosis.
BoTX: botulinum toxin; Chem: chemistry; LUTS: lower urinary tract symptoms; MS: multiple sclerosis; NO: nitrogen monoxide; OAB: overactive bladder; P/M: physiology or medicine; TeTX: tetanus toxin.
The impact of the discovery of the endogenous neurotransmitters and neuromodulators, the characterisation of the transmitter receptor molecules, and the deciphering of intracellular signal transduction on drug development manifested in selected substance groups relevant in MS therapy.
While compromised cardiovascular function and temperature control remain to a large extent subject to individual adaptations in nutrition, clothing, and behaviour, disease-related dysfunctions of the pelvic organs are frequently approached by pharmaceutical intervention in addition to nutritional and behavioural adaptations. Classical antimuscarinic therapy has dominated treatment of overactive bladder syndromes, yet counterproductive effects on bowel activity as well as cognitive side effects remain a problem in addition to incomplete relief of the voiding symptoms. The application of BoTX to suppress neurotransmitter release may help in some cases.
The amazing success in the treatment of male sexual dysfunctions with PDE 5 inhibitors at the start to the 21st century sets new standards concerning efficacy and tolerability. Comparable success with respect to efficacy and tolerability of treatment in the case of bladder and bowel dysfunction would benefit not only both sexes of patients with MS, but also a large number of people affected by neurogenic and age-related disorders.