Abstract
Background: The pathophysiology of bipolar disorder is largely unknown; however, recent studies have suggested that metabolic dysfunction, particularly at the mitochondrial level, may represent a previously unexplored pathway. Lithium carbonate, valproic acid, and a combination of these represent the mainstay of treatment for bipolar disorder; however, the mechanisms underpinning the drugs’ clinical efficacy are not well characterised. At present, such mechanistic studies use concentrations which widely differ from the known bioavailability, thus, there is a need to establish the effect of lithium carbonate, valproic acid, and combination therapy at physiologically relevant doses.
Methods: Human astrocytoma 1321N1 cells were treated for 4, 24, and 48 hours. The MTT method was used to detect cytotoxicity upon drug treatment. Reactive oxygen species (ROS) production was quantified by dichlorofluorescin diacetate fluorescence.
Results: Upon H2O2-induced cellular stress, cell viability was significantly reduced; however, lithium exhibited a protective effect. In the absence of the stressor, the drugs had no negative effect on 1321N1 cellular viability. All the drug treatments exhibited protection against H2O2-induced ROS accumulation with lithium, bringing it closer to the control baseline.
Conclusion: The findings contribute to the understanding of the drugs’ biological effects, particularly as oxidative stress reducers. Furthermore, it highlights the need for research using comparable physiologically relevant models. This may advance the discovery of diagnostic biomarkers and new research approaches to the diagnosis of bipolar disorder.
INTRODUCTION
There is growing evidence for the connection between mitochondrial alterations in psychiatric disorders and psychiatric symptoms in mitochondrial disorders. There are high degrees of overlapping comorbidities and drug efficacies, suggesting that mood affective disorders incorporate components of mitochondrial dysfunction in their pathogenesis. Research has described mitochondrial structural abnormalities in patients with bipolar disorder (BPD) such as smaller surface areas and abnormal cellular distribution,1 mitochondrial DNA mutations, and polymorphisms,2,3 as well as widespread brain energy metabolism abnormalities.4 It is also common for bona fide mitochondrial disorders to be comorbid with psychotic symptoms (71% of patients5), which are often misdiagnosed as BPD and/or schizophrenia.6,7 All of these observations support the role of mitochondria in the clinical presentation of psychosis. Mitochondrial pathology can be a consequence of genetic susceptibility,7 dysregulation of neurotransmitter systems,8 or environmental insults such as exposure to famine, infections, toxins, or substance abuse,9,10 all of which are also risk factors for BPD.11
Bipolar Disorder
BPD is a chronic mood affective disorder characterised by episodic behavioural disturbances of mania (or hypomania) and depression. The current International Classification of Diseases (ICD) nosological classification of the disorder divides it into BPD type I (BPD-I), BPD type II (BPD-II), cyclothymic disorder, and recurrent manic episodes not otherwise specified (NOS).12 While BPD-I is the most severe type, with alternating episodes of mania and depression which often lead to hospitalisation, BPD-II is characterised by hypomania and depression.12,13 The lifetime prevalence is 0.4–1.0% for BPD-I and 3.0–4.0% for BPD-II.13 When compared to the general public, a person with BPD has a 20-fold higher risk of suicide.13 Individuals with BPD often require long-term drug treatment aimed at preventing relapses and recurrences. In the UK, lithium, valproic acid (VPA), olanzapine, and quetiapine are the main therapeutic drugs used for BPD.14
Lithium
Lithium is a mood stabiliser, used as a prophylactic agent in BPD as well as in the management of manic or hypomanic episodes. It is a naturally occurring water-soluble ion which does not bind to plasma proteins and is characterised by linear pharmacokinetics (proportional amount of drug administered and its blood concentration). Only lithium carbonate (LICA) and lithium citrate integrate the British National Formulary (BNF). Although the bioavailability of the two preparations is similar,15 peak lithium concentration is 10% higher in LICA preparations16 thus, this is most widely prescribed.17 Lithium does not undergo metabolism and is almost exclusively excreted by the kidneys (renal clearance values of 10–40 mL/minute, strongly correlated with renal function).18 Lithium is able to cross the blood–brain barrier (BBB) and its brain concentration after therapeutic dosing has been reported to sit between 0.5–1.3-fold the serum concentration, peaking after 24 hours of administration.19-22 With that, brain concentrations at therapeutic LICA dosing sit between 0.20–1.30 mM; however, currently available in vitro studies have not significantly addressed this. For instance, Lopes-Ramos et al.23 used 2.50–7.50 mM LICA in pheochromocytoma PC12 rat cell culture, De-Paula et al.24 used 0.02–2.00 mM LICA, and Kurauchi et al.25 used 1.0–5.0 mM LICA in primary rat hippocampal neuronal cultures.
Valproic Acid
VPA is an anticonvulsant drug, generally used in the treatment of epilepsy. In addition to this, VPA has other clinical indications, including BPD, given its mood-stabilising properties. The absorption of VPA is complete and rapid from the gastrointestinal tract, reaching peak plasma concentration within 4 hours of oral tablet administration.26 Its metabolism is complex and the majority of the administered dose is excreted in urine in the form of various metabolites.26,27 Unlike lithium, VPA binds well to plasma proteins (>90%), particularly albumin.26,28 This binding is saturable, with free VPA fraction higher when total plasma concentrations are elevated.28 The unbound portion is considered pharmacologically-active and able to cross the BBB, with reports of brain to serum ratios varying from 0.068–0.540 after VPA administration.29-32 As such, it is estimated that, at therapeutic doses, brain concentration sit between 20–325 μM; however, available in vitro studies have not actively addressed this: Tan et al.33 used 1 mM VPA in primary rat cortical cells; Wang et al.34 used primary rat astrocyte cells and 0.3–1.2 mM VPA; and Zhang et al.35 used human glioblastoma U87 cell line and 2–16 mM VPA.
Thus, the aim of this study was to investigate the putatively protective effects of physiologically relevant concentrations of LICA and VPA on cytotoxicity and oxidative stress in astrocytoma 1321N1 cells. Findings from this study are the first to identify the biological effects of these drugs, alone and in combination, on astrocytoma cellular biology using physiologically relevant doses.
METHODS
Reagents
LICA, VPA, and all other chemicals were procured from Sigma-Aldrich (Haverhill, UK), unless otherwise specified. All chemicals were of high commercial grade.
Cell Culture
1321N1 (human astrocytoma) cells were cultured in complete growth medium made from Dulbecco’s modified Eagle’s medium high glucose GlutaMAX™ plus 10% (v/v) fetal bovine serum and 1% (v/v) penicillin-streptomycin. The cells were maintained at 37 °C in a humidified 5% carbon dioxide atmosphere. Approximately 1×104 cells were harvested per assay and incubated for 24 hours in complete growth medium before proceeding. Cells from passage 39–49 were used.
Drugs
LICA (Li2CO3, 73.89 g/mol) and VPA (C8H15NaO2, 166.19 g/mol) were resuspended in water and filter sterilised before use. LICA stock was prepared at a concentration of 10 mM at room temperature, whereas VPA stock was 3 mM and stored at 4 °C, as per the manufacturer’s recommendations. The drugs were warmed to 37 °C for successive experiments. The physiologically relevant working concentrations of LICA and VPA were selected on the basis of previously mentioned pharmacokinetic evidence.19-22,29-32
Morphology Studies
Cell morphology was monitored at various time points during drug treatment by obtaining brightfield microscopy pictures using the ZOE™ Fluorescent Cell Imager (Bio-Rad). All images were obtained at 175x magnification.
Measurement of Cell Viability
To evaluate the effects of LICA and VPA on cell viability levels, 3-(4,5-dimethylthiazol-2-yl)-2,5-diphenyl tetrazolium bromide MTT assays were used. Cells seeded in 96-well plates for 24 hours were treated with 100 µL/well of treatment and control solutions. The cells were exposed for different periods of time (4, 24, and 48 hours) before 40 µL MTT (5 mg/mL) were added to each well. The cells were then incubated for 2 hours at 37 °C, followed by the addition of 80 µL dimethyl sulfoxide (DMSO) to solubilise the purple formazan crystals. The amount of dye released from metabolically active cells was measured at 620 nm with a microplate reader (Tecan Sunrise™) immediately after DMSO addition. Viability was determined by dividing the absorbance of treated cells by that of untreated (vehicle control) cells.
Quantification of Intracellular Reactive Oxygen Species Levels
Intracellular ROS production was assessed using a ROS-sensitive fluorescent probe, 2’,7’-dichlorofluorescin diacetate (DCFDA). Briefly, cells were incubated in black-walled 96-well plates with DCFDA (10 µM/well) for 30 minutes, in dark conditions and at 37 °C. The fluorescent dye-loaded cells were washed once in Dulbecco’s phosphate-buffered saline before the addition of 100 µL/well of treatment and control solutions. ROS production was detected as an increase in fluorescence, using the VICTOR™ X3 microplate reader (PerkinElmer) set to do 1-second reads/well at 485 nm excitation and 535 nm emission.
A Model of Cytotoxic and Oxidative Stress Conditions
1321N1 cells were exposed to two concentrations of H2O2 (120 and 480 µM), which were co-administered with LICA and/or VPA and incubated for 24 hours prior to MTT or DCFDA assay. This dismutated, non-radical, semi-stable ROS served as stimuli mimicking physiological cytotoxic and oxidative stress conditions in BPD.36,37
Statistical Analysis
Unless otherwise specified, all data is expressed as mean ± standard error of the mean (SEM). Data from at least three independent triplicate experiments was analysed using GraphPad to give n=1. Grubb’s outlier test was used for all studies to establish any significant outliers. The variance in data sets was analysed using the Mann–Whitney test followed by the T-test. For three or more groups, variance was assessed by using Bartlett’s test with data sets not reaching significance studied by Kruskal–Wallis test followed by Dunn’s test. Significance was reached when p<0.05.
RESULTS
Lithium Carbonate and Valproic Acid have no Effect on Cell Viability Following Exposure in 1321N1 Cells
The effect of physiologically relevant concentrations of LICA and VPA on cell survival was firstly investigated. MTT analysis indicated that after exposure, neither 1.3 mM LICA, 325 μM VPA, nor their combination (COMBO) significantly affected 1321N1 cell viability (Figures 1a, 1b, and 1c). At 48 hours of exposure, there is a trend for cell viability decrease with COMBO treatment; however, it is not statistically significant (Figure 1c). This is further evidenced by the accumulation of debris observed with brightfield imaging (Figure 1d). At 48 hours, untreated 1321N1 cells are non-confluent and well-organised in layers; however, upon LICA treatment numerous cells appear rounded, withered, disconnected, and floating in the media (Figure 1d). Upon VPA treatment, there is a noteworthy observation of distinctively thinner and longer cells at 48 hours of treatment, which may be indicative of cytoskeletal changes rather than cell death-related pathways (Figure 1d). Therefore, use of these drugs at physiologically relevant concentrations does not affect cell viability but do appear to impact on cellular morphology.
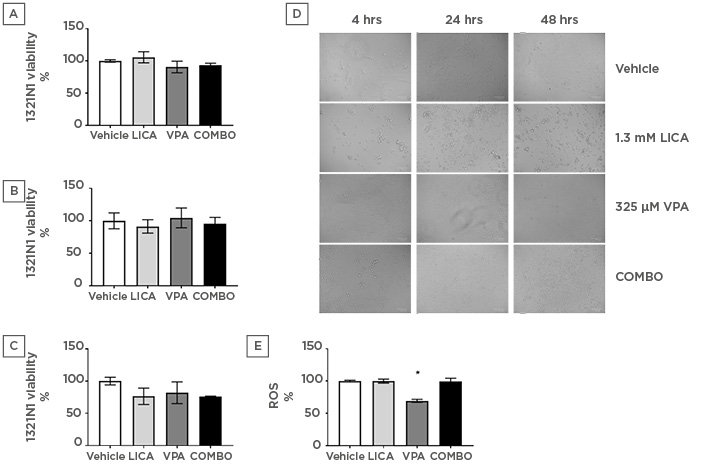
Figure 1: Physiologically relevant concentrations of valproic acid, but not lithium carbonate, significantly reduce oxidative stress in astrocytoma cells.
1321N1 astrocytoma cells were exposed to LICA (1.3 mM), VPA (325 µM), or a combination of the two (COMBO) for 4 (a), 24 (b), and 48 (c) hours. A-C: Cell viability was assessed by MTT assay. D: Morphology was assessed by microscopy with brightfield images. Scale bar represents 100 µm, magnification 175x. E: Oxidative stress was assessed by ROS accumulation using DCFDA dye for 1 hour. Data are presented as mean ± S.E.M with n of 3-4. Data were analysed using one-way ANOVA and compared to vehicle control with significance observed for ROS accumulation in VPA-treated cells compared to vehicle-treated cells. *p<0.05 versus vehicle for LICA and VPA. DCFDA: 2’,7’-dichlorofluorescin diacetate; LICA: lithium carbonate; MTT: 3-(4,5-dimethylthiazol-2-yl)-2,5- diphenyl tetrazolium bromide; ROS: reactive oxygen species; VPA: valproic acid.
Valproic Acid, but not Lithium Carbonate, Decreases Reactive Oxygen Species Accumulation in 1321N1 Cells
The next study investigated the mechanism through which this cellular stress could be regulated. The possibility that LICA and VPA generated free radicals was assessed using the DCFDA assay. During the time of incubation, it was shown that VPA altered baseline intracellular ROS production by significantly reducing it from 100.0±1.3% to 69.3±2.3% (Figure 1e). Conversely, neither LICA (99.8±3.1%) nor COMBO (99.6±4.7%) affected DCFDA fluorescence (Figure 1e). Thus, VPA exposure, but not LICA or a combination of therapies, decreases ROS accumulation in human astrocytoma cells.
Lithium Carbonate, but not Valproic Acid or a Combination Treatment, Significantly Protects 1321N1 Cells from H2O2-Induced Cell Death
Using H2O2 as a physiological cellular stress model,36,37 the effect of LICA and VPA on cell viability was assessed. At 480 μM H2O2 treatment, the three drug treatments displayed significantly reduced 1321N1 cell viability, being unsuccessful at restoring cell viability compared to their respective drug-only counterpart (Figure 2a). Only LICA significantly protected 1321N1 from cellular death when compared to H2O2-only treatment (Figure 2a). Cell viability in the absence of LICA was 65.6±0.8% for 120 µM H2O2 and 50.1±1.7% for 480 µM H2O2. In contrast, in the presence of LICA, cell viability was maintained near baseline at 94.8±2.3% for 120 µM H2O2 and 71.6±3.4% for 480 µM H2O2. Notably, COMBO did not produce any statistically significant protective effect when compared to H2O2-only treatment or its drug-only equivalent (at 120 μM H2O2). Microscopically, an apparent increase in cell number is present upon drug treatment when compared to H2O2-only (Figure 2c). This cellular stressor also triggers cell ballooning ameliorated by all treatments tested presently (Figure 2c).
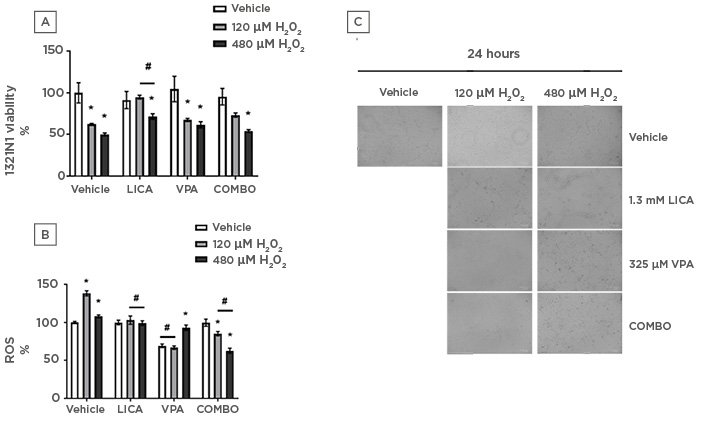
Figure 2: Physiologically relevant concentrations of both lithium carbonate and valproic acid attenuate oxidative stress in astrocytoma cells whilst only lithium carbonate protects against H2O2-induced cell death.
1321N1 astrocytoma cells were exposed to LICA (1.3 mM), VPA (325 µM), or a combination of the two (COMBO) in the presence and absence of hydrogen peroxide (120 and 480 µM H2O2) for 24 hours. A) Cell viability was assessed by MTT assay. B) Oxidative stress was assessed by ROS accumulation using DCFDA dye for 1 hour. C) Morphology was assessed by microscopy with brightfield images. Scale bar represents 100 µm, magnification 175x. Data are presented as mean ± S.E.M with n of 3-4. Data was analysed using two-way ANOVA and compared to vehicle control for drugs or vehicle for H2O2. Significance was observed for cell viability and ROS accumulation when treated 120 and 480 µM H2O2 versus the vehicle. *p<0.05 versus vehicle for H2O2. Significance was also observed in cell viability and ROS accumulation measurements for LICA-treated and COMBO-treated cells with H2O2 co-treatment (120 and 480 µM) versus vehicle-treated cells with H2O2 co-treatment (120 and 480 µM H2O2). Significance was also observed for ROS accumulation studies for VPA-treated cells treated with vehicle or 120 µM H2O2 versus the vehicle for the drug. #p<0.05 versus vehicle for LICA and VPA.
Lithium Carbonate, Valproic Acid, and their Combination Significantly Attenuate H2O2-Induced Reactive Oxygen Species Accumulation in 1321N1 Cells
To evaluate the effect of LICA and VPA on the H2O2-induced formation of ROS, DCFDA fluorescence was assayed. LICA significantly reduced ROS production compared to H2O2 treatment, bringing it to a comparable level to its drug-only counterpart thus exhibiting a protective effect (Figure 2b). Conversely, VPA and its co-treatment with 120 μM H2O2 significantly reduced ROS and are comparable to each other (Figure 2b). Interestingly, VPA co-treatment with 480 μM H2O2 resulted in significantly higher ROS build-up than drug-only but close to the 100% baseline. These findings may relate to the relative DCFDA fluorescence of 1321N1 cells, given their significantly decreased viability upon said co-treatment (Figure 2a). That said, there was a decrease in cell viability from 65.6±0.8% in 120 μM H2O2-treated cells to 50.1±1.7% following exposure to 480 μM H2O2. This was coupled with a decrease in ROS accumulation from 138.3±3.4% in 120 μM H2O2-treated cells to 108±1.9% following exposure to 480 μM H2O2. Lastly, COMBO was effective at reducing ROS production both when compared to H2O2 and drug treatment (Figure 2b). Taken together, these findings demonstrate that, at physiologically relevant concentrations, all three drug treatments attenuate ROS build-up with LICA being most effective at returning it to a vehicle-comparable baseline.
DISCUSSION
The long-term management of BPD is complex. Both LICA and VPA are recommended by the National Institute for Health and Care Excellence (NICE) as first-line treatment and, indeed, these are the most commonly prescribed drugs for long-term maintenance.38 In the present study, the cytotoxic effects of LICA and VPA at physiologically relevant concentrations were investigated in human astrocytoma 1321N1 cells. The present findings demonstrated that in the absence of cellular stressors, none of the presently tested drug treatments affected cellular viability at 4, 24, or 48 hours (Figures 1a, 1b, and 1c). Furthermore, only VPA significantly affected intracellular ROS accumulation, reducing it below baseline levels (Figure 1e). The findings were consistent with the proven safety of these drug treatments, making them the most commonly prescribed medication for BPD.14
The present study used a generally unreactive endogenous ROS, H2O2, to mimic physiological conditions in BPD. This ROS is present in very low intracellular concentrations under normal physiological conditions but can considerably increase via feed-forward systems under pathological settings.36,37 The cell viability study demonstrated that only LICA significantly restored cell viability when compared to H2O2 (Figure 2a). Nevertheless, all drug treatments significantly ameliorated cell ballooning triggered by H2O2 (Figure 2c). This may be indicative of a time-dependent response to the stressor which has not been investigated in the present study. Furthermore, a growing body of research has demonstrated that oxidative damage from ROS is involved in neuropsychiatric disorders.39 Previous studies have demonstrated that astroglial cells are more susceptible to oxidative stress because of poor antioxidant protection.40 To test the protective effect of physiologically relevant concentrations of LICA and VPA on H2O2-induced oxidative stress, intracellular ROS in 1321N1 cells was monitored by DCFDA assay. At 120 μM H2O2, H2O2-induced ROS was significantly reduced by the three tested drug treatments (Figure 2b). This suggests that these treatments could also be effective against downstream targets of ROS responsible for astroglial death such as lipid peroxidation.40 This, allied to the reduced ROS production, would be in accordance with inhibition of oxidative stress and prevention of cell damage. However, the mechanism through which this may occur remains inconclusive in this study, as it may be a result of free radical-scavenging activity (for instance, catalase, glutathione peroxidase, or superoxide dismutase), altered mitochondrial membrane permeability, or a combination of these. At 480 μM H2O2, H2O2-induced ROS was significantly reduced by LICA and COMBO (Figure 2b). In these conditions, although VPA reduced ROS levels, this was not significant when compared to H2O2-only treated cells (Figure 2b). Furthermore, COMBO not only significantly prevented ROS build-up caused by 480 μM H2O2 co-treatment, it also reduced this accumulation below its drug-only baseline. This unexpected finding likely correlates to the reduction in cell viability present in this treatment (Figure 2a).
Previous studies have shown that H2O2 regulates the balance between bcl-2 and bcl-2-associated X protein (Bax), altering mitochondrial membrane permeability, activating caspase cascades, and upregulating p53, ultimately leading to apoptosis.11,40 Furthermore, the bcl-2 family of regulator proteins plays an important role in apoptosis and cellular injury during neuropsychiatric pathology progression, including BPD.11 The process of apoptosis is characterised by morphological changes including decrease in cell volume and chromatin condensation in the nucleus. The morphological findings of treatment with LICA and, to a lesser extent, VPA, could be indicative of this (Figure 2c). Further studies should aim to confirm whether the treatments were inducers of apoptosis in 1321N1 cells, by use of staining agents such as Hoechst 33342.41 Such methodology would allow for determination of the suggestive apoptogenic effect. Another noteworthy observation was the presence of dark intracellular inclusions after COMBO treatment and, to a lesser extent, VPA (Figure 2c). These could be significant misfolded protein aggregations, which often underlie the toxicity associated with neurodegenerative and neuropsychiatric disorders.42
Additionally, the findings in this study should be contemplated within the context of practical limitations. The 1321N1 cell line is commonly used in basic molecular and cellular biology research relating to astroglial cell function43,44 but is derived from U-118MG cells which are human malignant glioblastomas.45 The oncogenic modifications associated with such may have altered cellular regulation processes, differing from normal astroglial cells.46,47 Yet, immortal cancerous cell lines such as 1321N1 are often used in research as they provide several advantages such as being cost-effective, easy to use, and providing a virtually unlimited supply of materials, whilst bypassing ethical concerns associated with animal and human testing.46 They do, however, hold the limitation of suffering genotypic and phenotypic variation over serial sub-culturing events, therefore meaning they may not adequately represent primary cells.46 Nevertheless, cell culture models are well-established, frequently used tools in both basic research and drug testing.43,44,48 To surpass any limitations, further in vitro work could aim to confirm the findings using primary brain endothelial cells43,44 or immortalised brain-endothelial co-cultured cell lines, two recognised BBB models for neuropharmaceutical screening.47,49 Ultimately, to accurately address the organisational sophistication and myriad interactions between systems in the whole organism, in vivo studies would be necessary.
Due to the heterogeneity of BPD and lack of understanding of its pathophysiology, there is no specific biological marker which diagnoses the disease, thus current clinical practice relies on studying the patient’s medical history and thorough behavioural assessment.50 It is possible that the increasingly available research, such as that presented, will lead to a new focus on BPD within which the disease is seen as metabolic leading to new research approaches to treatment and diagnosis. Importantly, said research should take into consideration the pharmacokinetic evidence available on these drugs, addressing physiologically relevant hypotheses such as that presented.
CONCLUSION
In summary, based on the data provided by the present study, it has been demonstrated that physiologically relevant concentrations of LICA, VPA, and COMBO do play a role on the metabolic hypothesis of BPD by affecting cell viability and ROS production thus further elucidating their clinical efficacy mechanisms. It remains to be explored the exact mechanisms by which these protective effects act on 1321N1 cells; however, this study draws attention to the different trending hypotheses that view BPD as systemic and requiring different clinical approaches as well as further research addressing treatment resistance and mechanisms of efficacy.