Abstract
Cardiovascular disease is a leading cause of death and disability worldwide. Current treatment strategies aimed at treating the consequences of coronary artery disease have embraced both optimal medical therapy and catheter based percutaneous coronary intervention with drug-eluting stents (DES). Current-generation DES elute predominantly mammalian target of rapamycin (mTOR) inhibitors, which act primarily as a cytostatic agent that retards vascular smooth muscle cell proliferation and migration; this occurs in response to injury and thus prevents restenosis. While DES have reduced restenosis, the use of first-generation DES was associated with an increased risk of late stent thrombosis and accelerated neointimal atherosclerosis (i.e. neoatherosclerosis), both major contributors to late stent failure. The underlying substrate of late DES failure is likely related to vascular endothelial dysfunction, which occurs after DES implantation. Initial concerns with first-generation DES have led to improvements in stent design, polymer load and biocompatibility, and pharmacologic agents, all of which have helped to improve healing responses, lessen late stent failure, and result in an overall improved safety profile. The armamentarium of DES has expanded from the current-generation durable polymer DES to bioresorbable polymer DES, polymer-free DES, and lastly totally bioresorbable vascular scaffolds with a goal of improving vascular responses and endothelial function while preserving anti-restenotic efficacy. We will review these contemporary DES in relation to their short and long-term effects on vascular biocompatibility and healing responses.
INTRODUCTION
Drug-eluting stents (DES) are endovascular devices designed to prevent restenosis through inhibition of smooth muscle cell proliferation that is incited by vascular injury from initial device deployment.1 Since their introduction into clinical use, major advances have occurred in the DES design involving thinner metal scaffolds with preserved radial strength, more biocompatible polymers, and advancement in mammalian target of rapamycin (mTOR) inhibitor analogues, allowing for better drug retention in the vessel reducing the total amount of drug load needed per device. Each improvement in these DES components has, for the most part, improved the vascular responses to current-generation devices, lessening the delay in re-endothelialisation of stent struts and reducing the thrombotic risk. Despite these improvements, overall signs of vascular dysfunction still occur in current-generation metallic DES including inhibition of normal coronary vasomotion (largely due to the encasement of the vessel within a metallic cage) and the accelerated development of collections of foamy macrophages within the neointima (termed neoatherosclerosis). The latter may be due to incomplete endothelial junction formation and promotes the development of accelerated atherosclerosis leading to restenosis, late stent failure, and the need for target lesion revascularisation.2,3 This suggests that certain components in the DES system (e.g. the metallic backbone, mTOR inhibitors, and drug carrier polymers) may be the major contributors to endothelial dysfunction after DES deployment. Recent advancements in DES technology have aimed to reduce the vessel exposure to both carrier polymers, anti-proliferative agents, and even the metallic backbone itself. This has led to the development of newer endovascular devices such as the bioresorbable vascular scaffold (BVS), bioresorbable polymer DES (BP-DES), and polymer-free DES (PF-DES).4-7 Whilst current research into endovascular devices focusses on both metal backbone of the device itself as well as polymers used to deliver drugs from them, there have been advancements in the evolution of anti-proliferative agents for DES beyond limus-based derivatives. We will review current and future DES design and long-term risk for endothelial dysfunction and adverse clinical events.
CONTEMPORARY STENT DESIGN
Contemporary DES contain three primary components: a metallic backbone, polymer coating (which may or may not be absorbable), and lastly the anti-proliferative agent itself. For the sake of this discussion we will review the design of the three durable polymer DES (DP-DES) and one BP-DES currently approved by the US Food and Drug Administration (FDA) for human use. We will briefly touch upon the design of PF-DES and BVS systems, the latter of which was recently approved for clinical use in the USA. An overview of stent design elements by device type is shown in Table 1.
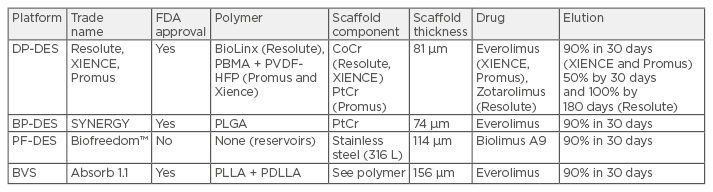
Table 1: Comparison of contemporary drug-eluting platforms.
DES: drug-eluting stents; DP: durable polymer; BP: bioresorbable polymer; PF: polymer free; PBMA: poly n-butyl methacrylate; PVDF-HFP: polyvinylidene fluoride and hexafluoropropylene monomers; PLGA: poly(lactic-co-glycolic acid); PLLA: poly-L-lactic acid; PDLLA: poly-D,L-lactide; CoCr: cobalt chromium; PtCr: platinum chromium; BVS: bioresorbable vascular scaffolds; FDA: US Food and Drug Administration.
Stent Platforms
Stent platforms currently in use in DP-DES, BP-DES, and PF-DES consist of cobalt nickel, cobalt chromium, or platinum-chromium alloys, providing an increased radial strength and radiopacity compared with stainless steel. All current-generation platforms are biocompatible and composed of thin struts (74–89 μm) which reduce inflammation and arterial injury and re-endothelialise at a faster rate than previous stents.
Polymers
Polymers applied to the surface of DES are designed to help control drug release. Polymers themselves can be immunogenic, generating hypersensitivity responses, which may result in delayed healing and in some cases stent thrombosis, as has been previously reported for both first and second-generation DP-DES.2,8 Even low-grade inflammations generated in response to polymers are thought to promote cell proliferation and intimal formation as has been shown in the porcine coronary model where first-generation sirolimus-eluting stents (SES) showed significant reduction in neointimal formation at 28 days compared to bare-metal stents (BMS) but this reduction was not sustained in the later time points.9 This was accompanied by evidence of increasing inflammation (i.e. granulomas and eosinophilic reactions) as well as cell proliferation, suggesting polymer induced inflammation as the cause of increasing intimal formation over time. This so-called late catch up phenomenon has been seen in both our human pathology DES registries as well as in clinical trials.10
The goal of polymer design for DP-DES has been to improve biocompatibility to help improve outcomes. Durable polymers such as polyvinylidene difluoride (PVDF) on the XIENCE™ Alpine (Abbott Vascular, Santa Clara, California, USA) and PROMUS Element™ (Boston Scientific, Marlborough, Massachusetts, USA) stents as well as the BioLinx™ polymer on the Resolute Integrity™ Stent (Medtronic, Minneapolis, Minnesota, USA). The BioLinx polymer consists of hydrophobic long-chain methacrylate esters, hydrophilic polar N-vinylpyrrolidone, vinyl acetate, and poly (n-butyl methacrylate), all of which have improved biocompatilibility compared to first-generation DES polymers. PVDF-coated stents had significantly lower thrombosis and platelet deposition compared with their bare-metal counterparts in experimental settings.11 In this regard, they have also been shown to elicit reduced platelet aggregates in blood contact applications.12 Whether other polymers used in DP-DES have similar effects remains incompletely explored.
The only FDA approved BP-DES in the USA is the SYNERGY™ stent system (Boston Scientific, Marlborough, Massachusetts, USA) which consists of a poly(lactic-co-glycolic acid) (PLGA) copolymer in which everolimus is released over the course of 3 months and the abluminal polymer layer becomes fully degraded by 4 months when PLGA biodegrades by hydrolysis of its ester linkages. Vascular responses were reported to be similar between SYNERGY, polymer-only stents, and corresponding BMS at 270 days in the porcine coronary model.13 These data reinforce the rationale for BP-DES wherein by eliminating long-term polymer exposure arterial inflammation is similar to BMS.
Bioresorbable Vascular Scaffold
BVS were designed to overcome the limitations of metallic stents including impaired coronary vasomotion and preclusion of bypass surgery for stented segments. The ability to provide transient scaffolding during repair of injured segments while minimising the risk of restenosis is the goal of BVS therapies. The development of the everolimus eluting Absorb™ (Abbott Vascular, Santa Clara, California, USA) BVS represents the most advanced attempt to bring such a system to clinical use. The bioabsorbable stent consists of a 150 μm thick poly-L-lactide scaffold with a poly-D-L-lactide coating. With the polylactic acid family of polymers molecular weight degradation occurs predominantly through hydrolysis, which includes a reduction in molecular weight first, followed by mass loss eventually leading to degradation of monomers (L-lactate) into pyruvate, which enters the Krebs cycle and is converted into carbon dioxide and water; practically speaking, the system takes 36–42 months to fully absorb. Long-term preclinical models utilising the Absorb system show promising results, but still raise interesting clinical questions. In the porcine model vascular responses to Absorb versus current- generation DP-DES (XIENCE V, Abbott Vascular) were comparable at all time points although inflammation scores and percent area stenosis were greater for Absorb versus XIENCE V from 6–36 months.7 In the rabbit iliac model of stenting, there was comparable endothelialisation of Absorb versus a first-generation DES (Cypher™, Johnson and Johnson, New Brunswick, New Jersey, USA) at 1 month with slight improvement at 36 months in addition to lower inflammation scores but greater area stenosis at the later time point.14 The greater area of stenosis was thought to be mainly due to its thicker struts. This suggests that BVS is least comparable to first-generation DES, however there are still concerns as to its readiness for clinical use compared with current-generation DES.15
Anti-Proliferative Drugs
DES utilise anti-proliferative agents to prevent restenosis. These anti-proliferative agents mainly consist of two classes: mTOR inhibitors and the taxol derivative paclitaxel. Initially first-generation DES utilised the mTOR inhibitor, sirolimus, in 2003 (Cypher, Johnson and Johnson), and soon after paclitaxel was used in 2004 (Taxus®, Boston Scientific, Marlborough, Massachusetts, USA). Since then the number of mTOR inhibitors has expanded in subsequent second and third-generation DES to become the predominant anti-proliferative agent eluted from these devices. Their use has extended into newer endovascular devices such as the BVS, BP-DES, and PF-DES.
The use of newer, lipophilic-limus based mTOR inhibitors (i.e. everolimus, zotarolimus, umirolimus [‘biolimus A9™’]) have also allowed lower drug concentrations lessening drug toxicity when compared to the prototype, sirolimus. While both sirolimus and everolimus have been used both systemically and locally, newer analogues (such as zotarolimus and umirolimus) have been specifically developed for local elution in vascular stents. The development of locally eluted sirolimus analogues has been initiated by the modification of the C40 or C42 moiety on the macrocyclic ring of the sirolimus backbone with a lipophilic group. In preclinical studies, zotarolimus with a tetrazole modification to C42 had the highest lipophilicity compared with sirolimus and paclitaxel, allowing for rapid vascular wall uptake and pharmacokinetic titration. In in vitro modelling of both endothelial proliferation and migration, sirolimus appeared to have more anti-proliferative and anti-migratory effects on endothelial cells versus everolimus.16
VASCULAR RESPONSES TO FIRST AND SECOND-GENERATION DURABLE POLYMER DRUG-ELUTING STENTS
Human pathologic studies looking at the vascular responses from patients with first-generation DP-DES demonstrated delayed arterial healing as defined by persistent fibrin, minimal neointimal formation, and incomplete endothelialisation in DP-DES compared to BMS cases at similar time points after stenting.8 Endothelialisation was complete in most BMS sections by 3–4 months while in first-generation DP-DES some samples remained unhealed as much as 40 months after implantation. Late stent thrombosis was defined as any platelet rich thrombus occupying 25% of lumen 30 days after DP-DES implantation, and was observed in >50% of patients receiving DP-DES.17 The major pathologic finding distinguishing late thromboses from patient DP-DES was evidence of a significantly greater delay in arterial healing characterised by lack of endothelialisation and persistent fibrin deposition at a mean of approximately 6 months after DES implantation.17 These data suggested that lack of complete arterial healing after DES was the common factor underlying all cases of DES late stent thrombosis. Further clinical data continued to demonstrate increased thrombotic events in patients receiving first-generation DES and indicated the most important risk factor for such events was withdrawal of dual antiplatelet therapy.18 Data from the SIRTAX and Post-SIRTAX registries in Bern and the RESEARCH and T-SEARCH registries in Rotterdam and Amsterdam indicated that stent thromboses continued to occur steadily, at a constant rate of 0.6% per year at least out to 4 years after stent implantation and perhaps beyond.19 Thus it seemed clear that in some patients receiving first-generation DES, arterial healing continued to be delayed for many years.
Second-generation DES such as everolimus-eluting stents (EES), Endeavor zotarolimus-eluting stents (E-ZES) (Medtronic), and Resolute Integrity zotarolimus-eluting stents (R-ZES) (Medtronic) were designed with thinner strut backbone stents, reduced polymer and drug loading, and eluted analogues of sirolimus such as everolimus and zotarolimus, which in some cases have improved lipophilicity as well as potentially increasing tissue retention and cellular targeting. In preclinical models of arterial stents, EES, E-ZES, and R-ZES demonstrate superior endothelialisation to first-generation DES at similar time points. In human autopsy samples of first-generation DES compared to second-generation DES (i.e. EES) EES demonstrated superior strut coverage at similar timepoints.2,8,20 Although head-to-head trials of first-generation DES (i.e. SES) versus EES have not conclusively shown a reduced incidence of stent thrombosis, all have probably been underpowered to reach such an endpoint. Optical coherence tomography (OCT) studies in patients receiving these stents have also been conducted and suggest superior strut coverage, a surrogate measure for endothelial coverage, in EES.21,22
VASCULAR RESPONSES TO ELIMINATING POLYMERS AND SCAFFOLD: BIORESORBABLE POLYMER DRUG-ELUTING STENTS, POLYMER-FREE DRUG-ELUTING STENTS, AND BIORESORBABLE VASCULAR SCAFFOLD
Despite evidence of improved endothelial recovery in second-generation DP-DES versus first-generation DP-DES, concerns about polymer induced inflammation as a cause of late catch up in both first and second-generation DP-DES prompted innovation in the design of metallic DES with the goal of limiting polymer exposure through either BP-DES or PF-DES. Of additional concern were rare reports of adverse effects of carrier polymers, such as hypersensitivity reactions with predominant eosinophilic infiltration and resulting intra-strut protrusions seen in OCT studies.23
Platforms involving BP-DES (SYNERGY) and PF-DES (Biofreedom™, Biosensors, Singapore) are in clinical use throughout the world though only SYNERGY is currently approved in the USA. Most BP-DES act similarly to DP-DES with less exposure of carrier polymer (degrades within 4 months) and similar efficacy.24 Vascular responses in the porcine model are similar between SYNERGY and control BMS, though SYNERGY showed mild increases in fibrin at early timepoints.13 Long-term inflammation was not reported in this particular study.
One particular issue of importance for BP-DES is how to show a definitive advantage in clinical endpoints versus second-generation DP-DES. In the EVOLVE II clinical trial of SYNERGY versus PROMUS (DP-DES), at 24 months, SYNERGY was non-inferior to PROMUS for target lesion failure (8.5% versus 9.4%, respectively). There were no significant differences in all-cause death (1.7% versus 2.1%), myocardial infarction (5.5% versus 5.3%), target lesion revascularisation (4.3% versus 3.1%), or stent thrombosis (0.4% versus 0.8%). It remains to be seen whether longer-term follow-up will demonstrate any clinically relevant differences. In the absence of data showing accelerated healing in SYNERGY versus DP-DES, dual antiplatelet duration is likely also to remain similar to current-generation DP-DES given similar drug elution characteristics (Table 1).
PF-DES also provides an interesting alternative combining the lack of polymer with the benefits of anti-proliferative drugs. Drug elution is through reservoirs within the metal scaffolding, which elutes the drug over a period of 30 days.25 With the Biofreedom PF-DES, studies have suggested that given this elution profile and absence of polymer, dual antiplatelet duration can be shortened to 1 month, similar to BMS with similar cardiovascular outcomes when compared to BMS in patients at a high risk of bleeding.25 In the porcine model, the Biofreedom stent demonstrated equivalent early reduction of intimal formation with improved late efficacy compared to the SES. At 180 days SES showed delayed healing and persistent inflammation compared with Biofreedom.26
One of the more innovative devices is the fully BVS which eliminates the long-term disadvantages of a metallic scaffold.7 Implantation of BVS is thought to restore the vasomotor integrity of the underlying vasculature seen in preclinical animal models.27 Because polymer is not as strong as metal, BVS has considerably thicker struts to improve its radial strength. Moreover, as stated earlier, polylactic acid degrades mainly by hydrolysis with complete degradation taking >32 months in animal models.7 In the rabbit model, endothelialisation, while similar to first-generation DES,14 is slower and more dysfunctional at 28 days as compared to DP-DES28 (Figure 1). A recent meta-analysis of BVS compared with current-generation DP-DES suggests that although target lesion revascularisation rates are equivalent, there is an increased risk of stent thrombosis compared with second-generation DP-DES (XIENCE).29 While BVS (Absorb, Abbott Vascular) provides a novel treatment of symptomatic coronary artery disease,29,30 it in many ways mirrors first-generation DP-DES in that the strut thickness is about twice that of current- generation DP-DES (Table 1) and thus it is not surprising that delayed healing is an issue with this device. BVS, like first-generation DP-DES, will likely undergo improvement in scaffold design leading to thinner struts with preserved radial strength.
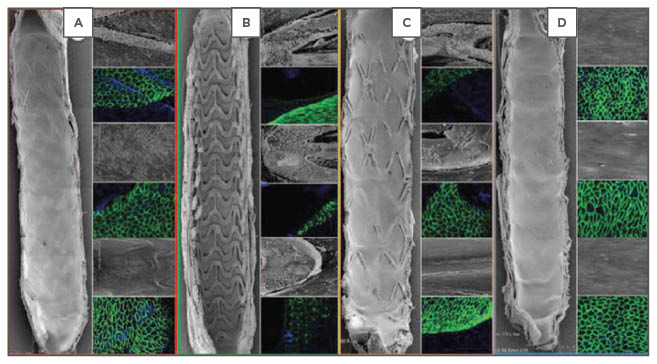
Figure 1: Representative images of endothelial coverage at Day 28 in a rabbit iliac artery model at 15x SEM (far right panel) and 200x SEM images (grey inset) and confocal microscopy (green stain) with endothelial protein staining (CD31/PECAM-1 expression).
A) Bioresorbable polymer everolimus-eluting stent; B) Everolimus-eluting bioresorbable vascular scaffold; C) Bioresorbable polymer biolimus-eluting stent; D) Bare-metal stent.
SEM: scanning electron microscopy.
While all these novel platforms provide different attributes compared to DP-DES, mTOR inhibitors remain a constant in all and its presence not only contributes to impaired initial endothelialisation but subsequent endothelial barrier dysfunction with acceleration of neoatheroma formation (i.e. neoatherosclerosis).
MECHANISMS OF IMPAIRED ENDOTHELIALISATION
Most current-generation DES used in clinical practice are designed to elute pharmacologic agents such as sirolimus that inhibit the mTOR, a member of the phosphatidylinositol kinase-related family of serine/threonine kinases. Although animal studies suggest that inhibitors of mTOR delay endothelial cell growth and recovery, the precise cellular mechanisms are still being elucidated.
mTOR interacts with several proteins to form two distinct complexes named mTOR complex 1 (mTORC1) and 2 (mTORC2), each of which has distinct sensitivities to rapamycin. Each mTOR complex integrates information from upstream signalling and activates downstream effectors to control distinct cellular mechanisms needed for arterial repair. mTORC1 is the better characterised of the mTOR complexes and integrates signalling from multiple signals including growth factors released upon arterial injury to affect process critical for endothelial coverage after injury such as migration and proliferation. The regulation of proteins critical (i.e. S6K1) for cell proliferation and migration might in fact be the most important mechanism by which mTORC1 regulates endothelialisation.3,16,25,31,32
Other factors also affect endothelial recovery. Local drug concentrations are increased by overlapping DES, encountered in approximately one-quarter of interventional procedures which may lead to an increased percentage of uncovered stent struts.33 However, the use of newer, lipophilic-limus based mTOR inhibitors (e.g. everolimus, zotarolimus) have also allowed lower drug concentrations, lessening drug toxicity when compared to the prototype, sirolimus. Furthermore, everolimus has been shown to have a more favourable vascular response in a preclinical diabetic animal model after DES implantation suggesting it may have a role in promoting endothelial integrity.5 Outside of pharmacological factors related to eluted drugs, various mechanical factors are related to poor endothelial healing and late stent failure. These factors include polymer hypersensitivity leading to eosinophilic infiltration, a rare but clinically important finding, and persistently poor endothelial healing due to either stent malapposition or underlying penetration of struts in necrotic core rich lesions.2 To some extent, these factors have been overcome by advances in stent technology in newer-generation DES with more biocompatible polymers and newer alloys and stent designs.
While improvements in design (i.e. mechanical and biological) factors may have largely addressed aetiologies of poor endothelial coverage after first-generation DES placement, an intact endothelium may display poor endothelial barrier function that may act as a substrate for neointimal atherosclerosis known as neoatherosclerosis (Figure 2). Neoatherosclerosis is the development of foamy macrophages within the neointima which overlies the deployed stent and is accelerated in DES compared with BMS. The use of limus-based DES may contribute to poor endothelial barrier function, leading to neoatherosclerosis, which is increasingly seen as a common substrate that underlies late stent failure leading to in-stent restenosis and thrombosis.
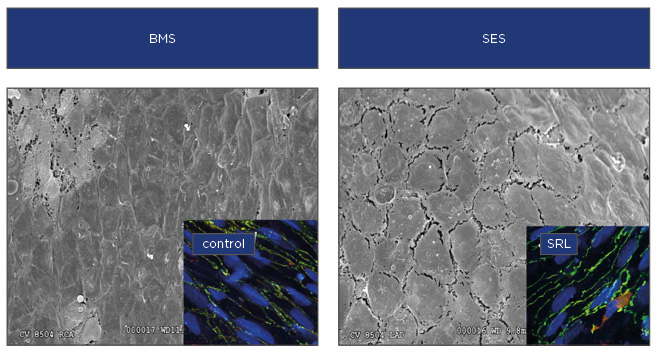
Figure 2: Poorly formed endothelial cell junctions following stent placement.
When compared with BMS, scanning electron microscopy of rabbit iliacs treated with SES have poorly formed endothelial junctions compared with BMS treated arteries and subsequent endothelial barrier dysfunction. Insets show immunohistochemistry of key endothelial barrier proteins with better formed junctions in control treated human endothelial cells compared with those treated with SRL.
BMS: bare-metal stents; SES: sirolimus-eluting stents; SRL: sirolimus.
NEOATHEROSCLEROSIS
Post-mortem studies of patients with late stent failure/stent related deaths have demonstrated both: i) poor endothelial coverage and ii) neointimal atherosclerosis (neoatherosclerosis) as common substrates of late sent failure. Key features of neoatherosclerosis include foamy macrophages, thin cap fibroatheroma, and lipid infiltration or plaque rupture. Accelerated neoatherosclerosis is seen with first-generation DES placement (mean ~420 days) and BMS (mean ~2,160 days) and may play a role in the greater observed incidence of late and very late stent thrombosis in DES versus BMS. We previously reported in an autopsy series of first and second-generation DES that the incidence of neoatherosclerosis was approximately 30% in both first and second-generation (i.e. EES) DES.2 There is a growing body of evidence that points towards the eluted limus-based agents as contributors to late events through a common pathologic pathway involving the accelerated neoatherosclerosis formation. The occurrence of accelerated atherosclerosis within DP-DES might be due to impaired endothelial barrier formation, which would allow transudation of lipid and immune cells into the arterial wall. We recently demonstrated that mTOR inhibitors can impair endothelial barrier formation both in cultured cells, mice, and human aortic specimens exposed to sirolimus ex vivo. We showed that sirolimus-FKBP12 interaction impairs barrier formation by increasing intracellular calcium via destabilisation of ryanodine intracellular calcium release channels and subsequent activation of calcium sensitive protein kinase C alpha (PKC-α), a serine/threonine kinase important in vascular endothelial cadherin barrier function through its interaction with a key endothelial junctional protein, p120-catenin (p120).3 This study demonstrated that the impairment in barrier formation that occurs after endothelial cells are treated with mTOR inhibitors occurs because of off-target effects of the drug itself rather than as a direct consequence of mTOR inhibition. These differences are likely exacerbated by diabetes where PKC activation is also associated with accelerated atherosclerosis suggesting that neoatherosclerosis is likely a major contributor to in stent restenosis, especially in diabetes. These data may also explain why the incidence of neoatherosclerosis is not different between first and second-generation DP-DES and will likely occur in newer stent platforms since all employ mTOR inhibitors.
While many believe BP-DES and BVS might obviate the formation of neoatheroma by eliminating vascular exposure to polymer, it has been observed clinically within the latter stent system.34 Overall it remains to be seen whether these newer stent systems alleviate the incidence of neoatherosclerosis.2
Recently we demonstrated a mechanism by which limus-based mTOR inhibitors inhibit endothelial barrier formation and likely contribute to neoatherosclerosis formation.3,16 We showed that sirolimus-FKBP12 interaction impairs barrier formation by increasing intracellular calcium via destabilisation of ryanodine intracellular calcium release channels and subsequent activation of calcium sensitive PKC-α, a serine/threonine kinase, important in vascular endothelial cadherin barrier function through its interaction with a key endothelial junctional protein, p120-catenin (p120).3 This study demonstrated that the impairment in barrier formation that occurs after endothelial cells are treated with limus-based agents occurs because of off-target effects of the drug itself rather than as a direct consequence of mTOR inhibition, suggesting that the different limus agents will likely result in similar rates of neoatherosclerosis. These differences are likely exacerbated by diabetes where PKC activation is also associated with accelerated atherosclerosis suggesting that neoatherosclerosis is a major contributor to in stent restenosis, especially in diabetes.35 These data may also explain why the incidence of neoatherosclerosis is not different between first and second-generation DES since all employ mTOR inhibitors which utilise similar mechanisms (i.e. FKBP12) to inhibit mTOR.2 Although neoatherosclerosis has been observed with BVS, future studies will elucidate the overall incidence compared with current- generation DP-DES.
CONCLUSION
Current-generation DP-DES and newer DES platforms (BP-DES, PF-DES, BVS) have the advantage of long-term patency rates (i.e. low target vessel revascularisation) with suppression of restenosis and an overall low rate of in stent thrombosis. Newer platforms attempt to obviate risk by eliminating the polymer, removing the metal scaffold in BVS and using newer lipophilic mTOR inhibitors. While current clinical data suggest newer platforms are non-inferior to DP-DES, their potential advantages have yet to materialise and may depend on a unique clinical situation. While PF-DES may have a definite use in high-risk bleeding patients, the advantage of BP-DES and first-generation BVS compared with current-generation DP-DES has not yet been fully elucidated. BVS may have the greatest therapeutic potential as compared to DP-DES because they offer the potential of restoration of normalising vasomotion and vessel remodelling.27 The main concern with BVS is its structural design, with thicker stent struts and compromised radial strength preventing complete endothelialisation and inadequate stent expansion. This has led to an overall observed increase in stent thrombosis in first-generation BVS. Further improvement in novel stent/scaffold design may likely lead to clinical improvements, however it may take long-term follow-up to elucidate reduction in target lesion revascularisation and reduction in long-term vascular effects such as neoatherosclerosis.