Abstract
Extracellular vesicles (EV) are subcellular components derived from a cell that are essential mediators of intercellular communication. Exosomes are a type of extracellular vesicle secreted by the cell. Exosomes have immense therapeutic significance and benefits in a variety of medical applications, including medication nanodelivery, cell and gene therapy, immunological response, biomarker therapy, and anticancer vaccination.
Methods for isolating exosomes, including ultracentrifugation, size-based isolation, immune-affinity capture-based approaches, precipitation, and microfluidic methods, are described in this brief review. Additionally, studies that demonstrate the diverse potential of exosomes as agents in diagnosis and natural agent therapeutics are briefly discussed.
The potential of exosomes extends to anticancer vaccines, with evidence supporting their role in inducing potent antitumor immune responses. Despite advancements, further research is needed to unlock the full diagnostic and therapeutic capabilities of exosomes. In summary, this concise review highlights the multifaceted nature of exosomes, showcasing their crucial role in intercellular communication and their diverse applications in biomedicine.
Key Points
1. Avoiding immune responses to delivery vehicles during delivery of natural and biologic drugs is of paramount importance, unlike the delivery of their synthetic counterparts.2. This article summarises the potential of extracellular vesicles, including exosomes, as natural cellular ‘couriers’ of biological information.
3. Affordable and accessible sources of extracellular vesicles vary in the biological world, and may be modified according to the needs of its application, for example with genetically engineered donor cells.
INTRODUCTION
Intercellular communication, whether through direct cell–cell interactions or the transfer of secreted chemicals, is critical for the survival of multicellular organisms. The physiologic processes of the human body are regulated by three distinct organ systems: the neurological system, the immune system, and the endocrine system.1
Cell–cell interaction is important because it:
a) regulates the metabolism and other functions of the tissue’s constituent cells;
b) serves as a communication channel that enables the coordination of cellular differentiation and proliferation in both embryonic and adult tissues; and
c) functions as a component of cellular recognition.
Cells spontaneously release endosomal and plasma membrane-originated vesicles, which are called extracellular vesicles (EV). EVs are made up of a fluid core surrounded by a lipid bilayer. Compared to its origin i.e., the donor cell membrane, the lipid bilayer of EVs is more rigid because it is primarily enriched by desaturated lipids such as gangliosides and sphingomyelin.2 These vesicles usually contain heat shock proteins, cytoskeletal proteins, and cytosolic DNA from secreting cells in abundance.3
Transmembrane proteins, integrins, and glycoproteins involved in vesicle trafficking cover the EV.4 EVs have a significant function in cell–cell communication by transporting membrane and cytosolic proteins, lipids, and RNA molecules between cells. Additionally, they contribute to synaptic transmission and hormone release through vesicular exocytosis.These vesicles are commonly known by various names such as macrovesicles, ectosomes, shedding vesicles, or microparticles.5-7 The term “exosome” was originally coined for vesicles with a diameter ranging from 30–100 nm, which are ‘exfoliated’ by various cultured cells or their natural tissues.8 Subsequently, it was discovered that exosomes are also secreted into circulation by B lymphocytes and dendritic cells using a similar mechanism through cellular membrane fusion of micro-vesicular bodies (MVB) to be released outside cell (Figure 1).9,10
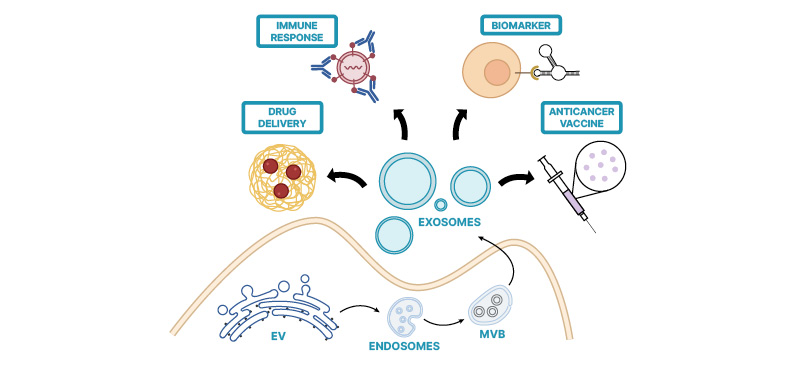
Figure 1: Biogenesis of Exosomes.
EV: extracellular vesicles; MVB: micro-vesicular bodies.
Exosomes, minute extracellular vesicles essential for intercellular communication, undergo a sophisticated biogenesis predominantly within the endosomal system. The process initiates with inward budding of the plasma membrane, forming early endosomes, which subsequently mature into MVBs through endosomal membrane invagination. Within MVBs, intraluminal vesicles form via the inward budding of the limiting membrane. This intricate process relies on the orchestrated action of various proteins, including endosomal sorting complex required for transport (ESCRT) machinery, lipid-modifying enzymes, and tetraspanins. Upon fusion of MVBs with the plasma membrane, intraluminal vesicles are released into the extracellular space as exosomes. Cargo sorting into exosomes is meticulously regulated and encompasses proteins, lipids, nucleic acids, and metabolites, mirroring the parent cell’s physiological state. The biogenesis of exosomes is pivotal in numerous biological processes, such as immune modulation, cancer advancement, and neuronal signalling, underscoring the imperative to comprehend its molecular intricacies.3,4,11
CHARACTERISTICS OF EXOSOMES
Characteristics of the exosomes can be seen in Table 1.12
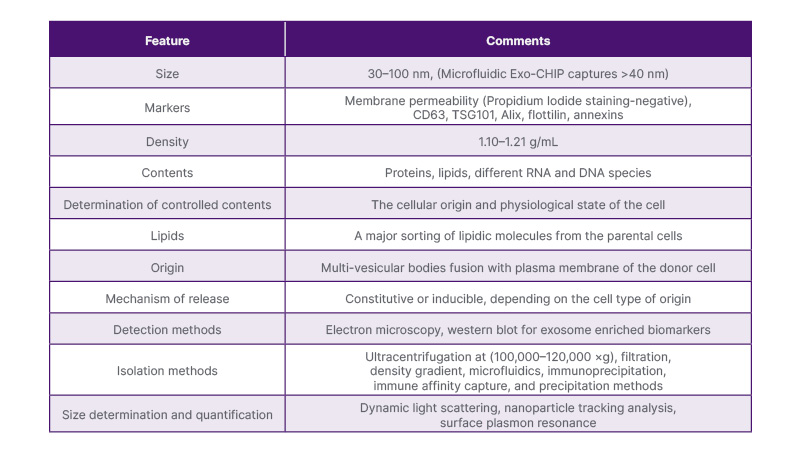
Table 1: Characteristics of exosomes.12
METHODS FOR ISOLATING EXOSOMES
Exosomes are small membrane vesicles released into the extracellular environment by various cell types. These vesicles can be isolated using the following methods:
Ultracentrifugation-Based Isolation Techniques
Centrifugation is a technique that employs centrifugal force to segregate mixed substances by exploiting the behaviour of particles in a centrifugal environment and their intrinsic properties such as mass, shape, and density. In the isolation of exosomes, a sequence of centrifugation cycles with varying forces and durations is utilised to separate them from other constituents in the sample based on differences in density and size.13
Ultracentrifugation typically employs forces ranging from approximately 100,000–120,000 ×g, for this purpose.14,15 Initially, a purification step is conducted to eliminate large bioparticles, and the sample is treated with protease inhibitors to safeguard exosomal proteins. Throughout the isolation process, supernatants are harvested intermittently, and either the supernatant or the pellet is resuspended, contingent upon the applied centrifugal force. Sequential centrifugation steps with escalating force are subsequently executed.16 Ultimately, the isolated exosomes are resuspended and stored at -80 °C until further examination. This method is alternatively known as the pelleting method or simple ultracentrifugation method. Ultrafiltration is thus a commonly utilised method for the isolation of exosomes based on size and/or molecular weight. The process separates suspended particles or polymers mainly according to their mass.
Exosome Isolation on the Basis of Size
Exosomes are first isolated through a series of filtration steps from cell culture supernatants. A standard 100 nm membrane filter is used to eliminate significant amounts of cell debris and floating cells, allowing flexible components larger than 100 nm to pass while removing rigid ones. Subsequently, tangential flow filtration employing hollow fibres with a 500 kDa molecular weight cutoff is applied to the filtrate, concentrating the retained material, followed by diafiltration to further purify the sample. A 100 nm track-and-echo filter is then utilised in the third step. Rinse cycles are incorporated after each phase to optimise exosome recovery, with continuous monitoring and maintenance of transmembrane pressure during the second and third filtration processes. Confirmation of exosome-associated proteins is achieved through mass spectrometry, alongside electron microscopy validating the vesicle morphology. The method’s gentle manipulation ensures high-purity exosome extraction while preserving functional integrity.17,18
Size exclusion chromatography is another technique employed for isolating exosomes based on size by utilising a porous stationary phase to separate macromolecules and particulate debris according to their size. Late elution occurs when components in the sample with small hydrodynamic radii can flow through the pores, while exosomes and other substances with larger hydrodynamic radii are hindered from passing through.19,20
Immunoaffinity Capture-Based Techniques
ELISA is a technique for analysing the antigen and antibody present in a given sample.21 The abundance of proteins and receptors on the exosome membrane creates an attractive opportunity for the development of precise isolation methods. These techniques hinge on immune-affinitive interactions between these membrane proteins (antigens) and their corresponding antibodies. Both qualitative and quantitative methods can gauge the levels of antigens and antibodies. Ideally, exosome biomarkers suitable for immune-based isolation should be firmly anchored to the membrane, lacking soluble forms, and either exclusively expressed or highly concentrated on the exosome surface from specific biological origins.22 ELISA outcomes, commonly represented as absorbance values, facilitate swift comparisons of known exosome surface biomarker expressions, thus offering immediate insights into exosome yield and specificity.23
Exosome Precipitation
Exosomes may undergo different degrees of solubility or dispersion, leading them to separate from biological fluids. Water-excluding polymers like polyethylene glycol (PEG) are employed for this purpose. These polymers bind water molecules, thus prompting less soluble components to precipitate out of the solution.24 Typically, solutions for precipitation containing PEG with a molecular weight of 8000 Da are mixed with samples and incubated. The resulting precipitate containing exosomes is then isolated through low-speed centrifugation or filtration after overnight incubation at 4 °C.25 This method of exosome precipitation is straightforward and does not require specialised equipment. With current technologies, it can be easily integrated into clinical settings and is adaptable for processing large sample volumes.26
Microfluidics-Based Isolation Techniques
This leverages both the physical and biochemical properties of exosomes at the microscale, benefiting from rapid advancements in microfabrication technology. These advancements offer a distinct opportunity to create microfluidic devices capable of efficiently extracting exosomes. These devices employ innovative sorting methods, including auditory, electrophoretic, and electromagnetic manipulations, alongside traditional techniques like size, density, and immunoaffinity. Such devices are expected to significantly reduce sample volume, reagent usage, and isolation duration.27,28 Additionally, this technique allows for precise dynamic control over analyte concentrations during sample processing and analysis.29 Furthermore, it enables manipulation of small sample volumes while maintaining high-throughput capabilities.
APPLICATIONS OF EXOSOMES
Drug Delivery Using Exosomes
Exosomes possess a remarkable capacity to transport beneficial cargo to diseased cells, making them highly promising therapeutic delivery systems on both theoretical and practical fronts. Their small size has been proven to be advantageous for delivering drugs to cancerous cells and neurons. Exosomes can be engineered to carry various therapeutic payloads, such as chemotherapeutic drugs, immunological modulators, antisense oligonucleotides, short interfering RNAs (siRNA), and other agents. They can be sourced from a variety of origins including cow milk, macrophages, dendritic cells, and bone marrow.
For example, exosomes derived from RAW264.7 macrophages have been utilised to transport molecules targeting lung and breast cancer, as well as demonstrating effectiveness in treating Parkinson’s disease due to their high catalase concentration.30 Additionally, dendritic cells derived from the bone marrow of C57BL/6 mice offer reduced immunogenicity and have been employed to deliver siRNA to the brain in mice.31 Furthermore, exosomes from human bone marrow mesenchymal stem cells have been investigated for their ability to deliver functional anti-miR-9 antibodies to glioblastoma multiforme cells, serving as mediators of communication between mesenchymal stem cells and glioblastoma cells in the brain.32
The authors have compiled the resources used to isolate exosomes previously, and their prospective use as a medication delivery method (Table 2).31-34
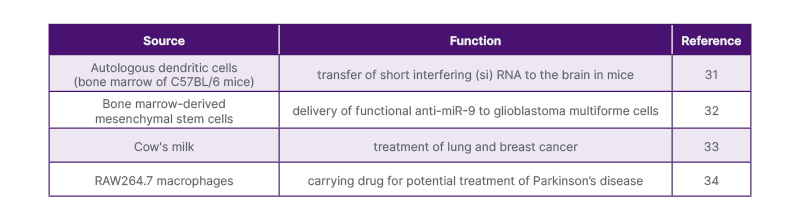
Table 2: Biological origins and function of exosomes.
All these studies show the potential of exosomes for use in the delivery of drugs and biologics. Small molecule medication loading is quite effective; however, loading DNA/siRNA still has room for improvement.
Immune Response
The exosome content has been found to be extremely disease-specific and includes information about cancer, viral infections, and neurodegenerative illnesses, including Alzheimer’s and prion diseases. However, in recent experiments, engineered exosomes have been shown to play a role in both innate and adaptive immune responses. This finding supports their potential for use in the development of immuno-therapies. The transfer and arrangement of antigenic peptides are responsible for the function of exosomes. The delivery of DNA that activates cyclic GMP-AMP synthase stimulator of interferon gene signalling to recipient cells occurs through an innate immune pathway. In this pathway, the detection of cytosolic DNA initiates the expression of inflammatory genes and triggers a Type I interferon response. This in turn may lead to exosomal miRNA-mediated gene expression regulation as well as the stimulation of a variety of signalling pathways by surface ligands.35
Exosomes play a role in preventing placental infection by delivering exosomal miRNAs, specifically the chromosome-19 miRNA cluster (C19MC), from specialised placental cells known as trophoblasts to cells outside the placenta. This mechanism helps safeguard the placenta against viruses like poliovirus, human cytomegalovirus, and herpes simplex virus-1.36
Exosomes present in breast milk play a crucial role in promoting post-natal growth and overall health. These exosomes contain immune-related miRNAs and have been shown to enhance the ex vivo generation of peripheral blood-derived T-regulatory cells. This function potentially aids in regulating immunological tolerance.37
Exosomes play a vital role in promoting viral infection. They disseminate viral components and promote their survival. For this reason, it is possible to deliver vectors containing genes of interest to target cells. Adeno-associated virus exosomes have been shown to effectively transfer genes to the immune system.38 This finding suggested that exogenous administration and endogenous exosomal secretion may trigger immunological responses in a context- and dose-dependent manner.
Exosomes as a Biomarker Source
Exosomes serve as a valuable source of biomarkers due to their critical role in facilitating cell–cell communication. They achieve this by directly interacting with surface ligands and transporting their contents between cells.39
Exosomes play a crucial role in transferring cellular mRNAs and microRNAs among cells.40 The analysis of the horizontal transfer of genetic information may help to investigate cancer recurrence and monitor responses to a certain therapy. The major advantage of using exosomes as biomarkers is that they eliminate the need for invasive methods. Moreover, these methods have been successfully used to detect ovarian, lung, and pancreatic cancers from liquid biopsies.41 Exosomes can remain stable and highly specific to target cells even after undergoing adverse conditions, such as numerous freeze‒thaw cycles and changes in pH.42
Even though exosomes and their components are used as biomarkers, these molecules cannot be used to quantify the severity of the disease. However, in the future, further research could identify exosomes as potential diagnostic, prognostic, and therapeutic delivery tools.
Potential Anticancer Vaccines
Understanding the mechanisms underlying antitumor immunity induced by exosome-based dendritic cell (DC) vaccines is crucial for determining the suitability of exosomes as tumour antigens in DC vaccine-based immunotherapy. Current research suggests that exosomes may indeed serve as optimal antigens for DC vaccines.43
DCs being the most effective antigen-presenting cells release numerous exosomes with potent anticancer properties. Exosomes derived from dendritic cells have been shown to induce activation in both CD4+ and CD8+ T cells containing key chaperones like major histocompatibility complex (MHC) I, MHC II, CD86, and HSP70-90.16,44 The transmission of exosomal peptide MHC I to CD8+ T cells is facilitated by the co-stimulation of released IL-2 and exosomal CD80. This process promotes CD8+ T cell proliferation, leading to a more robust antitumor immune response in vivo.45 Several studies have confirmed the activation of CD8+ and CD4+ T cells by exosomes derived from dendritic cells and the initiation of an in vivo antitumor immune response through exosomal CD80 and endogenous IL-2.46
CONCLUSION
Intercellular communication is pivotal for the coordination of physiological processes in multicellular organisms with neurological, immunological, and endocrine systems playing key roles. EVs emerged as crucial mediators in this communication network. Exosomes released by various cells exhibit unique characteristics, including a lipid bilayer enriched with specific and encapsulated cytosolic components for targeting specific tissues and their microenvironment.
Techniques based on size, molecular weight, immunoaffinity capture, ultracentrifugation, precipitation, and microfluidic approaches are employed for exosome isolation. These provide varying degrees of purity and efficiency, facilitating the exploration of exosomes for diverse applications. Exosomes, therefore, possess great promise in drug delivery, acting as carriers for therapeutic payloads such as chemotherapeutic drugs, RNAs, and immunomodulators. Their involvement in immune responses, both innate and adaptive, positions them as potential therapeutic tools. Additionally, exosomes serve as a source of biomarkers, enabling non-invasive detection of diseases, including cancer, and offering stability under adverse conditions.
The potential for exosomes as anticancer vaccines is a growing area of interest with studies demonstrating their ability to induce antitumor immune responses. While current research highlights their diagnostic and therapeutic potential, further investigations are warranted to fully comprehend the extent of their applications and their role in immunotherapy. In summary, exosomes present a multifaceted and promising avenue for advancing the understanding of intercellular communication and developing innovative biomedical applications in the future.