Meeting Summary
Mary Ellen Sanders opened the webinar by defining and differentiating the ‘biotic’ family, including probiotics, prebiotics, synbiotics, and postbiotics. She discussed the need for improved labels on commercial products in the biotics family and emphasised the research gaps in this field Ana Teresa Abreu expanded on a specific probiotic, Bacillus clausii, describing the evidence for health benefits associated with this bacterium and the potential mechanisms through which it might achieve these effects. Finally, Karine Clément discussed the role of the gut microbiome in cardiometabolic disease, suggesting that gut microbiota may represent a missing link between the environmental and genetic factors that impact these diseases. Clément described the evidence for a dysbiosis of gut microbiota in metabolic diseases and posited that a personalised approach to gut microbiome therapy might be the best way to leverage this association.
The Science of Probiotics and Related Biotics: How to Understand and Use Them
Mary Ellen Sanders
Mary Ellen Sanders introduced ‘biotics’ as a family of four microbiome-targeted substances: probiotics, prebiotics, synbiotics, and postbiotics. Each type of biotic has the potential to impact the resident microbes of a host, which have diverse physiological functions, including promotion of fat storage and angiogenesis, immune development, synthesis of vitamins and amino acids, drug metabolism, modification of the nervous system, breakdown of food, resistance to pathogens, protection against epithelial injury, and modulation of bone-mass density.1
Many human diseases and disorders are associated with an altered microbiome, including irritable bowel disease, colon cancer, diabetes, obesity, rheumatoid arthritis, and liver disease.1–3 However, Sanders emphasised that it is not yet clear whether the altered microbiome is a cause or a result of these conditions.1 This raises the question of whether restoring the microbiota in individuals with these conditions, to match that of healthy individuals, would affect the condition itself.
Biotics are intended to influence colonising microbiota to improve health, but understanding of what constitutes a healthy microbiome is still quite limited. Sanders explained that rather than focusing on the specific microbes present in the microbiome, a healthy microbiome may be better characterised by a high diversity of taxonomic units, high resilience (the ability to recover from perturbations such as antibiotic exposure), and functional redundancy (more than one ecosystem member can perform the same function).4
Sanders feels that although studying the microbiome is helpful to understand the mechanisms of biotics, the evidence of health benefits is more important. For example, probiotics have been shown to benefit health for various clinical endpoints, across the human lifespan, and in different organ systems, such as preventing antibiotic-associated or traveller’s diarrhoea, treating ulcerative colitis, and reducing the incidence of infection gastrointestinal disease.2 For most of these benefits, a microbiome-mediated mechanism has not been demonstrated yet.4
The International Scientific Association for Probiotics and Prebiotics (ISAPP) has published statements that include clear definition for each of member of the biotic family, based on consensus panels (Table 1). Importantly, these definitions are deliberately broad enough to support innovation; they do not restrict these substances by host (e.g., human, agricultural animals, etc), regulatory category (e.g., food, drug, or supplements), site of action (e.g., gut, vaginal tract, skin, etc), or mechanism of action.5-8
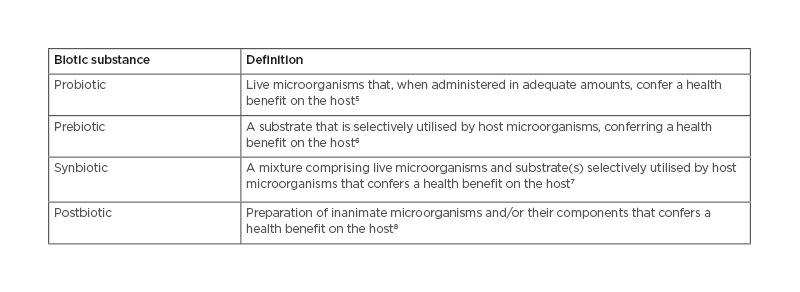
Table 1: ISAPP Definitions of biotic substances.
Definitions are concise; for full understanding, see the full statements. All substances must be safe for their
intended use.
ISAPP: International Scientific Association for Probiotics and Prebiotics.
Probiotics
A number of different microbes are used as probiotics, many of which are members of the Lactobacillaceae family or are species of Bifidobacterium, Bacillus, or Saccharomyces.9 The range of probiotic species is rapidly expanding10 as more is learnt about the microbes that reside in the healthy human body. Sanders emphasised the importance of recognising that probiotics are a heterogenous group; two products which contain the same microbial genus and species but differ by microbial strain may differ in function.
To be defined as a probiotic, a substance must be a properly identified (both sequenced and named). The microbe must be alive when administered, and studies need to have demonstrated a health benefit for a specific target host at the specific dose delivered by the product. In addition, the microbial strain and manufacturing process must be safe for the intended use, and the product must be correctly labelled with the strain and colony forming units (CFU) expected at the end of its shelf life.5
Ideally, probiotic product labels should detail health benefits (supported by evidence), suggested serving size, proper storage conditions, and contact details for consumer information.11 However, a survey of refrigerated probiotic foods in grocery stores in the USA found that only one-half (22 of 45) of products listed the constituent microbial strains. Those that did, could be linked to evidence of health benefits, tended to contain fewer strains, and had a lower CFU per serving compared to other products.12 A survey of probiotic supplements found similar results: most products could not be linked to evidence; 45% did not list constituent microbial strains; and 45% did not provide CFU at end of shelf life.13 Sanders emphasised that similar problems exist outside of the USA.
Neither probiotics nor postbiotics are required to target the microbiome directly, whereas prebiotics and synbiotics should do so as part of their mechanism of action.5–8 Despite these distinctions, Sanders explained that there is a common belief among both scientists and the general public that probiotics have an important impact on the gut microbiome. This belief is not fully substantiated by the available research data; a systematic review of clinical trials showed that probiotics did not have a global impact on the faecal microbial communities in healthy subjects.14 Sanders suggested that this does not prove that probiotics have no effect; their effects may be limited to minor components of the microbiota, not evident in faecal samples or in healthy subjects, or only evident in the metabolites rather than the microbiome composition. However, she stressed that the evidence to date indicates that the effects of current probiotics on the microbiome are likely to be quite subtle.
In summary, Sanders reiterated that the healthy gut microbiome has not yet been defined by researchers, but that for probiotics, effects on the microbiome are probably less important than health benefits. There is a clear need for improved labels on commercial products in the biotics family so that healthcare practitioners and consumers know what they are buying, and the terms probiotics, prebiotics, synbiotics, and postbiotics should only be used when the scientifically accepted criteria are met. She emphasised the research gaps in this arena, including defining a healthy microbiome, robust trials to confirm health benefits, and identifying the best strains and doses for specific applications. Finally, Sanders emphasised that it will be important to understand the mechanisms that drive the clinical benefits of biotics in order to optimise these substances for future use.
Bacillus clausii: Mechanisms as Spore Probiotics in Gastrointestinal Disorders
Ana Teresa Abreu
Bacillus is one of the most studied bacterial genera15 and its species can be found in soil, water, food, and in the human gut.16 These aerobic bacteria can differentiate into a dormant endospore, allowing them to survive in stomach acid and bile salts in the gastrointestinal system.16,17
Most Bacilli are not pathogenic to humans or animals, and in the case of B. clausii (Figure 1),¹⁸ an endosymbiotic relationship, where one organism lives inside the other, between species and their hosts has been suggested.17,19,20 Four strains of B. clausii are resistant to antibiotics, a property considered advantageous to restoring a healthy gut, and are named for their predominant antibiotic resistance: novobiocin and rifampicin (strain N/R), chloramphenicol (strain O/C), streptomycin and neomycin (strain SIN), and tetracycline (strain T). 19
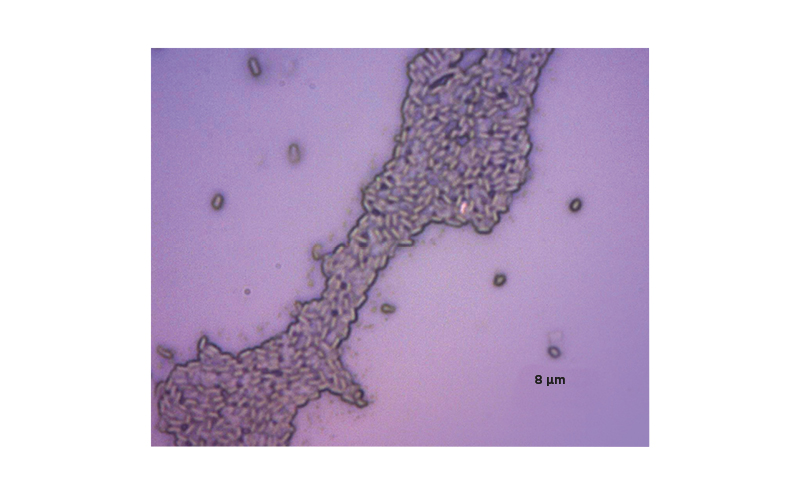
Figure 1: B. clausii (combined antibiotic resistant strains: O/C, SIN, N/R, T).
N/R: novobiocin and rifampicin; O/C: chloramphenicol; SIN: streptomycin and neomycin; T: tetracycline.
There are several properties of B. clausii that contribute to its probiotic effects:
- B. clausii spores can survive the hostile environment of the gastrointestinal tract and multiply to colonise the intestine.21-23
- The pan-genome of B. clausii (O/C, SIN, N/R, T) includes genes involved in carbohydrate metabolism,19 and one strain, SKAL 16, has been shown to excrete butyrate in in vitro conditions.24 Butyrate serves as the major energy source for enterocytes, exerts anti-inflammatory effects, and enhances gut barrier function.25
- The antibiotic resistance genes of B. clausii are stable and cannot be transferred to other bacteria.26 Many strains of B. clausii are recommended for use along with antibiotics, and Abreu emphasised that it is important for clinicians to match probiotic strains to the prescribed antibiotic therapy.
- Some strains of B. clausii, particularly SIN and T, produce the essential vitamin riboflavin (vitamin B2) in vitro, suggesting that B. clausii has the potential to compensate for host deficits in riboflavin that can occur in clinical contexts such as chemotherapy.27
- Bacillus species produce a wide range of antimicrobial substances, including lantibiotics (post-translationally modified peptides) which are active against gram-positive bacteria such as Clostridium difficile.20,28 One such lantibiotic, clausin, has been isolated from B. clausii and interacts with lipid intermediates in the bacterial envelope biosynthesis pathways,29 suggesting that it could help to manipulate the constituents of the intestinal microbiota.
Immunomodulation
B. clausii has been shown to have immunomodulatory properties in preclinical studies. In a human enterocyte model of rotavirus infection, B. clausii strains (O/C, SIN, N/R, and T) induced the synthesis of bacteriocins, reduced enterocyte cell death, and inhibited the release of pro-inflammatory cytokines. They also increased mucin production and the synthesis of tight junction proteins, both important for the integrity of the gut mucosal barrier.30 In addition, a small in vivo experiment has shown that B. clausii modifies the gene expression profile in the intestine in patients with mild oesophagitis, including genes involved in immunity and inflammation.31 Finally, in an animal model of asthma, B. clausii reduced the numbers of eosinophils, neutrophils, and lymphocytes, and lowered IL-4 and IL-5 levels, suggesting a potential use in reducing airway inflammation in clinical settings.32
Abreu explained that one potential mechanism for the immunomodulatory capacity of probiotic B. clausii strains could be the expression of extracellular compounds and/or immunostimulation via the cell wall. In murine cell lines, B. clausii MTC 8326 was shown to activate metabolic activity and innate immune responses in macrophages,33 and B. clausii (O/C, N/R, SIN, and T) was also shown to stimulate the production of nitrite in peritoneal cells, IFN-γ in spleen cells, and CD4+ T-cell proliferation.20 One route through which B. clausii may induce these immunomodulatory effects is through the secretion of lipoteichoic acid.34
Gut Homeostasis
Other studies have suggested that B. clausii contributes to gut homeostasis. In an in vitro simulation of the human gastrointestinal tract, B. clausii SC 109 spores (along with other probiotic bacteria and prebiotic ingredients) were shown to increase microbiome production of butyrate, and the overall diversity of gut microbiota.35 The presence of B. clausii in patients with pancreatic adenocarcinoma has been associated with longer survival times,36 and treatment with B. clausii UBBC07 has been shown to reduce serum urea levels in rats with acetaminophen-induced renal failure, suggesting a novel clinical use for probiotics in chronic kidney disease.37
Antimicrobial Properties
Abreu explained that B. clausii can produce antimicrobial peptides, including lantibiotics, that inhibit the growth of pathogenic bacteria in vitro.20 This characteristic means that probiotics can be supportive when delivered alongside antibiotic therapy. B. clausii (O/C, N/R, SIN, and T) appears to be protective during Escherichia coli infection in mice, increasing protective mucus secretion and resulting in minimal mucosal damage and less sloughing of villus tips.38,39
Infection with C. difficile can result in symptoms ranging from diarrhoea to pseudomembranous colitis,40 and infection with B. cereus can cause vomiting, diarrhoea, and haemorrhage.41B. clausii strain O/C has been shown to secrete a serine protease capable of inhibiting the cytotoxic effects of both C. difficile and B. cereus in vitro.41
Abreau explained that B. clausii has been efficaciously and safely used in humans for several decades. For example, in patients with dietary endotoxemia, believed to be caused by disruptions in gut permeability, administration of probiotic strains including B. clausii was associated with a 42% reduction in post-prandial serum endotoxin and reductions in pro-inflammatory markers.42 In patients with recurrent aphthous stomatitis, a disease of the oral mucosa that results in ulcers and pain, local adjunct application of B. clausii, alongside glucocorticoid treatment, reduced oral pain and ulcer severity compared to glucocorticoid alone.43
In summary, Abreu reiterated that the physiological, antimicrobial, and immunomodulatory properties of B. clausii have been demonstrated both in vitro and in vivo; and antimicrobial activity against enteropathogens such as C. difficile and B. cereus has been demonstrated, providing one potential mode of action for the efficacy of this probiotic in gastrointestinal disorders. Further clinical studies using specific strains in targeted medical conditions are needed to validate these findings, and to increase the scientific credibility of B. clausii.
Gut Microbiota in Cardiometabolic Diseases
Karine Clément
Clément began by emphasising that there is a heavy societal burden from cardiometabolic and nutrition-related diseases and that the gut microbiota can be considered a ‘super-integrator’ for many of the risk factors for mortality.44
Obesity, the fourth highest risk factor for mortality in Western Europe,44 is associated with altered inter-organ cross-talk involving the intestinal tract, brain, adipose tissue, muscles, and others (PRIEST 2019). In the adipose tissue, obesity is connected to perturbed endocrine secretions, immune or inflammatory imbalances, altered angiogenesis, organelle dysfunction, altered extracellular matrix, and adipocyte hypertrophy.45–47 The development of obesity involves the pathogenic remodelling of white adipose tissue, which may lead to the development of obesity-related cardiometabolic disease and compromised response to obesity treatment.48 There is substantial heterogeneity in the clinical trajectory of subjects with obesity and their weight loss responses, for which gut microbiota-derived elements may be contributing factors.49
Clément explained that the role of the gut microbiota genomes in host biology should be considered: while it is accepted that both environmental and genetic factors play a role in the development of metabolic disease, gut microbiota may represent the missing link between them.
The key functions of the gut microbiota are in the digestion of food and the production of metabolites, the development and integrity of intestinal structure, immune system development, metabolism of toxic compounds, and synthesis of vitamins K and B.50 However, several studies have suggested that gut microbiota also play a role in energy balance and our capacity to store fat. Clément described ground-breaking pre-clinical experiments that showed that germ-free rodents have decreased adiposity and are resistant to diet-induced weight gain, compared to conventionally raised rodents.51 In addition, transplanting gut microbiota from mouse models of obesity into germ-free mice can partially transfer the obesity phenotype.51 Similar experiments have been conducted to transfer microbiota from humans to mice, and these have shown that the receipt of gut microbiota from an obese human can result in increased adiposity in a mouse, even when a healthy diet is followed. In parallel, the receipt of gut microbiota from a lean individual (a twin of the obese individual) results in a lean mouse when a healthy diet is followed52,53 (Figure 2).
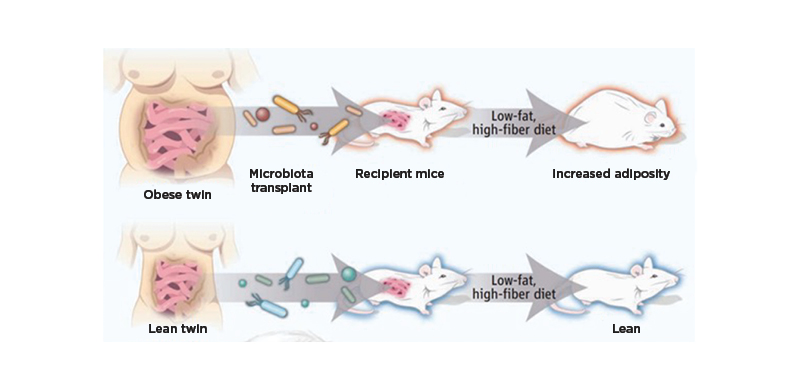
Figure 2: The protective role of gut microbiota from a lean donor in the presence of a healthy diet.
Reproduced with permission, Walker and Parkhill.52
Clément then discussed the importance of diversity in the gut microbiome in healthy individuals. Subjects living in westernised countries such as the USA have been shown to have a lower diversity of gut microbiota from an early age, compared to populations that are more isolated or live with an ancestral mode, such as Malawians or Amerindians.54 Some studies have attempted to stratify individuals by their microbiotic gene richness. Across these studies, 20–30% of subjects were considered to have low gene richness, and this group was characterised by increased overall adiposity, dysmetabolism, and a more pronounced inflammatory phenotype than individuals with high gene richness.55,56 Approximately 75% of patients with severe obesity (candidates for bariatric surgery) can be classified as having low gene richness. This is important because a low gene count is associated with enrichment of pro-inflammatory bacteria, whereas a high gene count is associated with enrichment of anti-inflammatory bacteria.55
One of the important characteristics of ‘healthy’ gut microbiota is the production of short-chain fatty acids (SCFAs), including butyrate.6,8,25,57 SCFAs act on enterocytes to stimulate the production of certain hormones, improving insulin sensitivity and glucose tolerance and modifying lipid metabolism.8,25 Clément emphasised that there is considerable research effort focused on understanding the imbalance between the gut microbiota in healthy individuals and those with disease. Gut microbiota may also contribute to the health of the intestinal barrier in metabolic diseases.58 For example, studies have shown that modification of the gut microbiota affects the thickness of the mucus barrier.58
The effects of gut microbiota on the host can be classified as metabolism-independent pathways, driven by components of the bacterial membrane such as lipopolysaccharide or peptidoglycan and impacting low-grade inflammation processes or modifying host biology; or metabolism-dependent pathways driven by microbial metabolites such as imidazole propionate, SCFAs, secondary bile acids, or trimethylamine. 59,60
Clément described several studies that have attempted to stratify gut microbiomes into groups based on their genome. In a European study, Arumugam et al., described three distinct clusters of microbiomes, termed enterotypes, each characterised by a dominant gut microbial species: Type 1, enriched in Bacteroides; Type 2, enriched in Prevotella; and Type 3, enriched in Ruminococcus.61 Subsequent studies have identified a subset of the Type 2 microbiome with a low proportion of Faecalibacterium and low microbial cell density, named Bact2, which is more prevalent in patients with inflammatory bowel disease versus the general population (78% versus 13%, respectively).62 The prevalence of Bact2 also correlates with higher BMI and with low-grade systemic inflammation in the MetaCardis European cohort.63
Clément explained that interventions to increase microbial diversity, increase beneficial microbes, and change metabolite concentrations are intended to improve metabolism and the immune response, potentially reducing the burden of complications. Potential mechanisms to modify the gut microbiome include dietary changes, selective enrichment of gut bacteria, faecal transplant, and bariatric surgery.
One example of such an intervention is diet-induced weight loss in patients with obesity or overweight, which improved gut microbiotic diversity and clinical phenotypes in patients with a low microbial gene count at baseline.56 Bariatric surgery also appears to increase microbial gene richness one-year post-surgery.63 Administration of Akkermansia muciniphila to mouse models of obesity or Type 2 diabetes resulted in a reduction in fat mass, insulin resistance, and low-grade inflammation,64 and A. muciniphila is associated with healthier metabolic status and greater insulin sensitivity in human subjects with obesity or overweight.65 Finally, a study of faecal transfer from healthy individuals to patients with obesity and metabolic syndrome showed an improvement in insulin sensitivity, however, the effect was transient and mainly observed in patients with a low gut microbiota diversity at baseline.66
Clément concluded that there is evidence for a dysbiosis of gut microbiota in metabolic diseases, and that a personalised approach to the gut microbiome may be the best way to leverage this association. However, she stressed that further research is needed as the links between the changes in the gut microbiota and the expected clinical effects have yet to be fully elucidated.
Summary
In summary, the evidence to date supports the hypothesis that both probiotics and the gut microbiome have an impact on the health of humans and other animals. However, though potential mechanisms of action have been suggested experimentally, further research including well-designed trials is needed to fully understand how probiotics manipulate the gut microbiota to benefit the host.
MAT-GLB-2104926