Abstract
Urinary tract infection (UTI) is one of the most common infections of childhood. The clinical presentations are mostly non-specific or mild. As any episode of UTI can potentially damage the kidneys, timely diagnosis and treatment are necessary to prevent renal damage. Incidence of UTI varies depending on the age, gender, and race of the child. UTIs in children are commonly caused by bacteria, though viruses, fungi, and parasites are also occasionally involved. The pathogenesis of UTI is complex where several host and pathogen factors influence the course of the disease and its outcome. Urine culture is still considered the gold standard method for the diagnosis of UTI. The means of obtaining urine samples from children for culture involves urethral catheterisation and suprapubic aspiration. The conventional methods of antibiotic susceptibility testing are labour intensive and time exhaustive. With the advent of technology, many automated platforms are available which are rapid, involve less volume of the culture or the sample, and have high accuracy.
INTRODUCTION
Urinary tract infection (UTI) is one of the most common infections afflicting all age groups, including children. Growing awareness has led to an increase in recognition of this disease entity and remains of immense importance, especially as it is known to present as an occult febrile illness in children, and with systemic symptoms, particularly in neonates. Despite the extensive information available, there are still some issues and challenges that need to be addressed. This article reflects on the epidemiology, pathogenesis, and laboratory diagnosis of childhood UTIs and provides a glimpse of some of the recent advancements in these aspects.
EPIDEMIOLOGY
Incidence of paediatric UTI varies depending on the age, gender, and race of the child. In the first 3 months of life, the incidence of UTI is higher in males than in females, with the male to female ratio of UTI being 2–5:1.1 With increasing age, the incidence of UTI among females increases hugely, and the male to female ratio becomes 1:10. Reports indicate that a considerable number of children experience at least one episode of symptomatic, culture positive UTI by the age of 5 years. The prevalence of febrile UTI is higher in white infants than in black infants, with the relative risk being 6-times higher in white infants.2 The initial presentation of UTI in children can be an occult, undiagnosed fever with or without mild gastrointestinal manifestation, or even upper and lower urinary tract symptoms.1 It is estimated that approximately 7% of children <2 years of age present with an idiopathic fever,2 with statistics demonstrating that the rate of febrile UTI is higher in uncircumcised boys compared with circumcised boys.3
AETIOLOGY
The most common causes of UTI in children are bacteria, though viruses, fungi, and parasites may also occasionally be implicated. Adenoviruses are notoriously associated with haemorrhagic cystitis. Candida, on the other hand, usually cause infection in immunocompromised children. The majority (80–90%) of childhood UTIs are caused by Escherichia coli, followed by other members of the Enterobacteriaceae family, namely Klebsiella, Proteus, Citrobacter, Serratia, and Enterobacter.4,5 Within these, Proteus mirabilis has an affinity towards the balanopreputial sac where it remains colonised and hence, is frequently encountered in male children. Among the Gram-positive bacterial pathogens, which are encountered less frequently compared with the Gram-negative pathogens, Enterococcus and Streptococcus (Group B usually) are commonly isolated alongside other Gram-positive cocci. As Staphylococcus aureus rarely causes childhood UTI, incidences could be a result of haematogenous spread. The pathogens isolated and their antimicrobial susceptibility vary depending on whether the origin of UTI is community or hospital acquired.4,5
PATHOGENESIS
The pathogenesis of UTI is a complex interplay of several host and pathogen factors influencing the course of the disease and its outcome. The initial step is the essential colonisation of the periurethral region by the bacteria, without which they are washed out by the force of the urinary flow.6 The source for this is the large intestine where these organisms are present as normal commensals.7 Female anatomy predisposes to a higher risk of developing UTI; the issue arises as the periurethral region is in relatively close proximity to the anus, and also because the urethra is shorter in females compared with that of men. Hence, the most common route of UTI at any age is the ascending route, though rare haematogenous infections are known to occur.6
Following colonisation, the organisms express adhesion mechanisms/factors, which are best described and evaluated using uropathogenic E. coli (UPEC) as an example. UPEC have fimbriae, specialised rod-like appendages that protrude from the bacterial cell surface and secrete afimbrial adhesins, such as haemolysins, cytotoxic necrotising factors, and autotransporter proteins. Amongst these fimbriae, Type 1 and P fimbriae are highly important and are more often associated with cystitis and acute pyelonephritis, respectively. Besides all these, UPEC also possess capsules and lipopolysaccharides (LPS), which protect it from the complement-mediated killing and harmful effects of the cytokines, respectively.7 The host mounts immune defence mechanisms against uropathogens. There are a number of innate defence mechanisms that maintain the sterility of the urine within the urinary tract until the bladder neck. Beyond the bladder neck, urine can potentially be contaminated by the normal resident flora. A major defence mechanism against bacterial growth in the urinary tract is urination itself, which along with the shedding of surface epithelial cells, wards off any bacteria that may be adhered to them.6,8,9 Therefore, any obstruction to the flow of urine may lead to compromisation of this mechanism.
A fairly common entity encountered in young males are the posterior urethral valves. These valves predispose the child to repeated episodes of UTI and vesicoureteric reflux (VUR), which in turn can lead to reflux nephropathy followed by repeated episodes of pyelonephritis and renal scarring.10 VUR and renal scarring are more common in males than in females. Hence, extensive evaluation in males <2 years of age presenting with a UTI is required.5,10 The bladder mucosa is well protected by a layer of mucin secreted by the transitional epithelial cells lining the bladder, and this prevents bacterial adherence. Urine itself reduces the chances of the bacteria surviving as it is acidic and has a high content of urea, other salts, and organic acids. The mucosal immune response mediated by secretory immunoglobulin A also offers some protection against the bacterial infection as it is known to bind the bacteria and prevent their attachment to the urothelium. Some endogenous products are important mediators of the innate immune defence apparatus. One such substance is the Tamm–Horsfall protein. This is secreted in the ascending limb of the loop of Henle and behaves as an immunomodulator, activating cells of the immune system, namely granulocytes and macrophages. This activation prevents the colonisation of the urothelium by uropathogens using Type 1 fimbriae.7,8 Binding of the Type 1 fimbria activates the secretion of the other endogenous substances such as antimicrobial peptides, specifically defensins. There are two types of defensins, and , both of which are bactericidal. -defensins are released by neutrophils, while -defensins are released by the renal epithelium.11
The presence of toll-like receptors (TLRs) on the epithelial lining allows them to bind to the pathogens by recognising their surface molecules. This initiates a signalling cascade, which activates an immune response. The TLRs present on the urothelium include TLR2, which can recognise the peptidoglycans in the cell wall of Gram-positive bacteria, and TLR4 which can recognise the LPS present in the cell wall of Gram-negative uropathogens.8,12,13 Another recently identified TLR, TLR11, has a putative, but not yet established, role in the pathogenesis of UTI wherein it is said to be capable of recognising the uropathogens. In this way the latter offers protection to the kidney from bacterial damage.14 The binding of the fimbriae to the specific TLR4 triggers a cascade of various inflammatory mediators releasing cytokines and the activation of complement.12,13 This immune activation causes inflammatory changes leading to the recruitment of neutrophils and macrophages at the site of inflammation, and ultimately culminating in a scar formation, as well as clearing off the bacterial infection. Thus, the scar formation at sites of inflammation in the renal parenchyma is a fallout of this inflammatory cascade rather than a direct insult due to the pathogen.12
Circumcision is known to reduce the incidence of UTI in young males. The rate of UTIs in circumcised boys has been estimated to be around 0.2–0.4%, while the rate in uncircumcised boys has been estimated to be around 1–8%.15 Other than age, gender, and race, the genetic composition of an individual also influences the occurrence of UTI e.g. non‑secretors of the P blood group antigen are inherently at risk of developing a UTI.16
When given for a long time, broad‑spectrum antibiotics (e.g. cephalexin, cefixime) can alter the normal microbiota present in the gastrointestinal tract and periurethral region, thereby increasing the risk of UTI. Studies have shown that when antibiotics were prophylactically given to control asymptomatic bacteriuria, the chance of UTI increased.17 In a recent randomised, double-blind, placebo-controlled trial, RIVUR (Randomised Intervention of Children with Vesicoureteral Reflux), it was shown that antimicrobial prophylaxis among children with VUR substantially reduced the risk of recurrence of UTI, but did not reduce renal scarring.18 The pathogenesis of UTI requires a well-coordinated and orchestrated series of all of these factors in order to begin the disease process; a better understanding of these factors is necessary for its control.
SAMPLE COLLECTION
The ideal sample for the laboratory diagnosis of UTI is urine. Urine should be collected before the initiation of antibacterial therapy, as a single antibiotic dose can be the cause of a sterile urine culture. Sampling of urine from children is a challenging task. The means of obtaining urine samples from children involves urethral catheterisation or suprapubic aspiration.17,19-21 The sampling of urine using a collection bag or pad, though non-invasive, is unreliable as it is associated with a high contamination rate, which poses major diagnostic dilemmas. In toilet-trained children, a midstream urine sample is preferable.2
SAMPLE PROCESSING
Urine should be processed as soon as the sample is received in the laboratory. It is best to avoid any delay between the sample collection and processing. Since the generation time of E. coli is 20 minutes, this being the most commonly implicated organism in any UTI, any delay will reflect in the culture outcome as a false significant growth, which may not be the case. In cases where a delay is expected, the sample can be refrigerated for up to 24 hours at 4°C. The use of preservatives in the sample collection vials such as boric acid (1.8%), sodium chloride-polyvinyl pyrrolidone, and boric acid-glycerol-sodium formate can reduce the problems associated with the delay met during the transportation of urine samples.22 However, boric acid is usually associated with false negative results in dipstick testing.23
Macroscopic and Microscopic Examination
Macroscopic examination can provide crude indicators as to the presence of some pathologies. For example, turbid urine may indicate pyuria and red coloured urine may indicate cases of haematuria. However, these examinations are highly unreliable. Microscopic examination of urine can demonstrate the presence of pus cells, red blood cells, casts and bacteria, and yeast cells. The presence of seven or more pus cells per high power field in an uncentrifuged sample is suggestive of pyuria. On the other hand, the absence of pus cells does not reliably exclude a UTI, especially in infants <2 months of age.24,25 It is important to note that many infants with positive urine cultures but no pyuria probably have a contamination or asymptomatic bacteriuria rather than a UTI.26 It is now well understood that some paediatric patients with UTI can have normal urinalysis results at any given time.2 A Gram stain of unspun urine has a sensitivity and specificity approaching 90–95%; it has several advantages as it reveals the nature of the bacterium present and therefore guides the clinician to initiate antibiotic therapy. If a single bacterium is seen under one oil immersion field in a Gram stain, it is considered to be equivalent to 100,000 CFU/mL of bacteria (Table 1).27
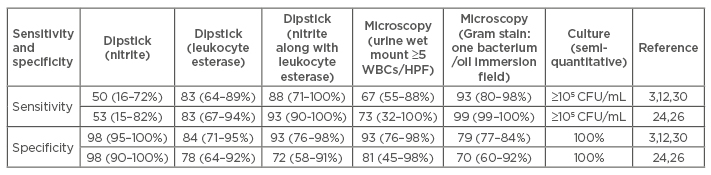
Table 1: Comparison of the diagnostic tests for UTI on uncentrifuged urine.
HPF: high power field; CFU: colony forming unit; WBC: white blood cell.
Rapid Tests for Screening
The conventional method of diagnosis by culture takes at least 24–48 hours, but rapid tests such as the dipstick test can reduce this delay. The dipstick test can detect nitrites and leukocyte esterase, and has a fairly good accuracy when compared with a semi-quantitative culture (Table 1).28 The nitrite test detects nitrite, which is produced from the metabolism of the nitrates by Gram-negative bacteria. In the case of infection with the Gram-positive bacteria, this test will be negative as these organisms do not convert nitrate to nitrite. Hence, the likelihood of a culture being positive is high due to a Gram-negative bacterium if the nitrite test is positive. If the contact time of urine with the bladder mucosa is <3 hours, this test can be falsely negative.
The leukocyte esterase test measures presence of pus cells in urine or pyuria. This test can be falsely negative if there are a low number of pus cells. A negative dipstick test for nitrite and leukocyte esterase and no pyuria or bacteriuria on microscopic examination has a high predictive value for the urine to be sterile on culture.27,29,30 However, this test can only be presumptive of UTI and cannot offer the antimicrobial susceptibility results, which is a major advantage with more conventional methods of diagnosis by culture.
Culture
Urine culture is the gold standard method for microbiological diagnosis of UTI.31 The most acceptable methods of isolation of pathogenic bacteria are the pour plate method and the semi-quantitative method, the former being resource and time exhaustive. To obviate the effect of the contamination due to the commensal microbiota that colonise the periurethral meatus, the concept introduced by Kass can be applied. Kass’ concept dictates that a threshold of 100,000 CFU/mL should be considered ideal for a culture to be positive in a midstream clean-catch urine sample.32,33 This concept holds true for asymptomatic children. It is the case that 10,000 CFU/mL is considered significant in symptomatic children and even less if the child has been catheterised. Where a sample is collected via suprapubic aspiration, any number of colonies should be considered significant.1,34,35 According to the American Academy of Pediatrics, abnormal urinanalysis (pyuria and/or bacteriuria) in the presence of 10,000 CFU/mL of a uropathogen isolated in culture is essential to establish a diagnosis of UTI.1,26,34
Most often UTI is monobacterial, unless there is a history of manipulation of the urogenital tract, obstruction (anatomical or otherwise) to urinary flow, or long-term catheterisation whilst receiving broad-spectrum antibiotics. It is important to distinguish true infection from contamination in cultures, and in cases where there is any uncertainty, a repeat sample should be requested. Asymptomatic bacteriuria produces a lot of confusion in children. Long-term studies involving children with asymptomatic bacteriuria showed that the majority of them spontaneously cleared the bacteriuria without treatment and only 2–4% of these infants went on to develop a symptomatic infection. None of the school-going children developed any symptomatic UTI.3,27,32
Antimicrobial Susceptibility Testing
Antibiotic resistance has become a universal phenomenon among bacteria, and hence, it is the moral responsibility of all diagnostic laboratories to perform susceptibility testing on all pathogens detected. Currently, laboratories across the globe either follow the Clinical Laboratory Standards Institute (CLSI) or the European Committee on Antimicrobial Susceptibility Testing (EUCAST) guidelines.36,37
Antibiotic susceptibility testing is either performed by disc diffusion testing, by the E-strip method, with commercially available automated systems, or with platforms for minimum inhibitory concentration (MIC) detection using the Vitek® (Biomerieux), the BD Phoenix™ Automated Microbiolog (Becton Dickinson diagnostics), or the MicroScan® WalkAway® (Siemens). The advantage of these commercially available systems over the conventional methods are that they are standardised with precise, ready-to-use platforms, based on the existing guidelines of either EUCAST or the CLSI and provide rapid reports of susceptibility.
Characterisation of resistance mechanisms are of epidemiological importance. The conventional methods available to detect these are labour intensive and time exhaustive. The detection of genetic determinants of antibiotic resistance using polymerase chain reaction (PCR)-based techniques (either conventional or real-time platforms) has revolutionised the field of antibiotic susceptibility testing. These platforms provide early information on antibiotic resistance to clinicians, as they have rapid turnaround times and can be directly performed on the clinical samples.38-40 Other methods such as the matrix-assisted laser desorption/ionisation time-of-flight mass spectrometry (MALDI-TOF MS) and microarrays have the advantage of being even more rapid and accurate. Further to this, they can be performed on very little volume of the analyte.41-43
MALDI-TOF MS can detect different mechanisms of antimicrobial resistance, for instance -lactamases, rRNA methyltranferases, etc. The spectrometer detects the peaks in the spectra generated by the hydrolysed and native unhydrolysed antibiotic molecule. The presence of a drug-hydrolysing enzyme is detected by the difference in the mass of the antibiotic molecule.41-43 The most recent inclusion into this list of revolutionary techniques is the microfluidics-based technique, also commonly referred to as the ‘laboratory on a chip’; a platform that originated from the combination of nanotechnology and bioengineering. This platform uses even smaller volumes of the analyte, which can be detected using optical, electrochemical, or magnetic devices.38,44-46 This involves the miniaturisation of the existing benchtop PCR thermal cyclers onto a disposable microchip. The small surface and apparatus allows rapid temperature alterations in very short reagent volumes, thereby reducing the total turn-around time compared to that taken by a conventional thermal cycler. When using a microfluidic device, it is easier to monitor bacterial growth in the presence of antibiotics and determine the minimum inhibitory concentration of the antibiotics. It is also quicker, taking a few hours rather than days. Some studies have estimated the electrochemical quantification of 16S rRNA levels, membrane potential changes in response to antibiotic induced stress, or the metabolites released, to measure bacterial growth in the presence of antibiotics.47,48
The most advanced method for determining the origin of antibiotic resistance is next generation sequencing, by which whole genome sequencing data can be analysed using bioinformatics. This technique has not been fully utilised due to its prohibitive cost and the extensive time required to sequence and analyse the whole genome of an isolated pathogen. Once the aforementioned limitations are improved upon, this will probably be the most robust technique available to detect and map antibiotic resistance routinely.38,49
CONCLUSION
It is important to consider UTI in a febrile child with non-specific, vague, or mild symptoms, especially in neonates, as UTI can lead to disastrous consequences if left untended. Therefore, any single episode of culture confirmed UTI should be considered important as a potential upper UTI and thus should be treated promptly.50