Abstract
Prostate-specific membrane antigen (PSMA) is the most extensively studied biomarker and antigen of prostate cancer. It is overexpressed in almost all prostate cancers, and the expression level increases with prostate cancer progression. PSMA is also highly expressed in the neovasculature of solid tumours including prostate cancer. As a result, numerous PSMA-specific ligands have been discovered for prostate cancer diagnosis and therapy, and one of them has been approved for clinical use. Moreover, a number of other PSMA-specific ligands are currently evaluated in clinical studies. In this review we discuss four major types of PSMA-specific ligands, including antibody, aptamer, peptide, and small molecule inhibitor. Their emerging applications in prostate cancer diagnosis, targeted drug delivery, and therapy are also discussed.
INTRODUCTION
Prostate cancer is the most common male malignancy and the second-leading cause of cancer death in American men. Current therapies for prostate cancer include surgery, chemotherapy, cryosurgery, radiation, and hormonal therapy. These approaches are used either as monotherapy or in combination according to stages in the patients. A variety of prostate cancer specific antigens have been explored for targeted drug delivery and diagnosis. Among them, prostate-specific membrane antigen (PSMA) is the most extensively studied biomarker and antigen of prostate cancer.1
PSMA, also known as folate hydrolase, N-acetyl-α-linked acidic dipeptidase I, or glutamate carboxypeptidase II, is a Type 2 transmembrane glycosylated protein. Belonging to the group of zinc-dependent exopeptidases with a binuclear zinc site, PSMA contains a glycosylated extracellular domain of 707 amino acids, a transmembrane domain of 24 amino acids, and an intracellular domain of 19 amino acids.1 The crystal structure of PSMA reveals a symmetric dimer with each polypeptide chain containing three functional and structural domains: a C-terminal/helical domain, an apical domain, and a protease domain.2,3 Although the exact physiologic function of PSMA in the prostate is not fully understood, it is known that PSMA can cleave g-linked glutamates from polyglutamated folates (known as folate hydrolase activity) and α-linked glutamate from N-acetyl aspartyl glutamate (known as NAALADase activity).4
PSMA is expressed in normal prostate epithelial cells, but its expression level in prostate cancer cells is much higher. Notably, PSMA is overexpressed in almost all prostate cancers, and the expression level increases with disease progression.1,5 Moreover, PSMA is overexpressed in the neovasculature of solid tumours, but not in normal tissue vasculature. PSMA expression is also detectable in several normal tissues, such as the small intestine, proximal renal tubules, and salivary glands,6 but the expression level of PSMA in prostate cancers is approximately 100 to 1000-fold higher.7,8 In addition, the site of PSMA expression in normal tissues (brush border/luminal location) is not exposed to direct blood circulation, leading to negligible interaction between PSMA-specific ligands and PSMA in normal tissues.1,8 As a result, PSMA has been recognised as the most valuable prostate cancer-specific antigen for prostate cancer diagnosis and therapy. The aim of this review is therefore to discuss the currently available PSMA-specific ligands (antibody, single chain fragment variable [scFv], aptamer, peptide, and small molecule) that have been explored for prostate cancer diagnosis and therapy (Figure 1).
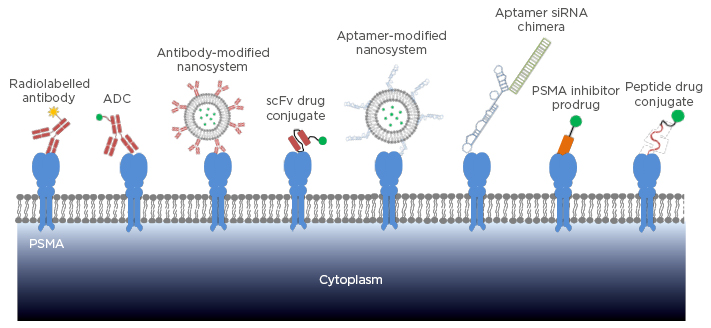
Figure 1: Targeting strategies of PSMA-specific ligands in prostate cancer diagnosis and therapy.
PSMA: prostate-specific membrane antigen; ADC: antibody drug conjugate; scFv: single chain fragment variable; siRNA: small interfering RNA.
PSMA-Specific Antibody and scFv
Antibody is by far the most common type of PSMA-specific ligand in preclinical and clinical studies. PSMA was initially identified by the murine monoclonal antibody (mAb) 7E11, which was discovered after immunisation of mice with preparations of PSMA-positive LNCaP cells.9 The mAb 7E11 has thereafter been used as the first anti-PSMA antibody for various prostate cancer treatments and diagnoses. For example, ProstaScint® (111Indium-labelled mAb7E11) was approved by the FDA for the imaging of prostate cancer.10,11,12 However, mAb 7E11 binds to the intracellular domain of PSMA and therefore cannot bind viable prostate cancer cells, thus limiting its potential clinical applications.13 Consequently, numerous efforts have been made to discover other mAbs targeting the extracellular domain of PSMA.
Using hybridoma technology, Bander and co- workers firstly discovered four mAbs (J59l, J533, J415, and E99) that specifically bind to the extracellular domain of PSMA with Kd values in the low nM range (1.76–18 nM).14,15 Later, these PSMA-specific mAbs were labelled with 131I and 111In for imaging studies in nude mice bearing LNCaP xenograft. These mAbs exhibit higher tumour uptake compared with radiolabelled 7E11. Moreover, 7E11 tends to accumulate in the areas of necrosis, whereas J591 and J415 mainly accumulate in viable tumours, suggesting the great therapeutic potential of mAbs in targeting the PSMA extracellular domain.16 Since then, several other groups have discovered numerous PSMA mAbs that target the extracellular domain of PSMA. All these mAbs show high affinity to purified PSMA as well as viable prostate cancer cells.17,18
Among the mAbs shown to target the PSMA extracellular domain, mAb J591 has attracted the most attention as an anti-PSMA antibody for prostate cancer diagnosis and therapy because of its high affinity and specificity to PSMA. Several J591-based imaging and therapeutic agents are currently being evaluated in clinical trials. For example, 89Zr-labelled J591 was developed as a positron emission tomography (PET) imaging agent to detect metastatic castration-resistant prostate cancer cells. The Phase I/II study reveals superior targeting of bone lesions compared with conventional imaging modalities or a combination of conventional imaging modalities.19 In another Phase II clinical trial, 177Lutetium-labelled J591 exhibits high tolerance, accurate tumour targeting, and dose-dependent prostate-specific antigen responses in metastatic castration-resistant prostate cancer patients, suggesting its promising potential of targeting cytotoxic agents to PSMA.20 177Lutetium-J591 in combination with docetaxel is in an ongoing Phase Iclinical study to evaluate its effectiveness against metastatic, castrate-resistant prostate cancer (Clinical Trial NCT00916123).
mAb J591 has also been conjugated to the cytotoxic agent monomethyl auristatin E through a valine-citrulline spacer to form an antibody drug conjugate (ADC).21 The J591-based ADC exhibits very high cytotoxicity (IC50 <0.022 nM) in PSMA-positive cell lines, but much less cytotoxicity (IC50 >30 nM) in PSMA-negative cells.21 The ADC dramatically reduces the size of docetaxel- refractory xenograft prostate tumours, including tumours larger than 700 mm3. Moreover, the survival rate of the ADC treated group is noticeably higher than that of the docetaxel treated group.21 The J591-based ADC is currently under evaluation in a Phase II clinical trial. In addition to radiolabelled mAb and ADC, mAb J591 was used as a targeting ligand in the PEGylated liposome loaded with alpha-particle generator nuclide 225Ac as the anti-cancer agent. The J591-labelled liposome specifically binds to several PSMA-positive cell lines and accordingly induces cell apoptosis.22
Wetterauer et al.17 discovered three other PSMA-specific mAbs (3/E7, 3/F11, 3/A12) from mice immunised with unpurified LNCaP lysate. These mAbs demonstrate high affinity to purified PSMA, viable LNCaP cells, and PSMA-transfected cells. Further studies showed that these three mAbs bind to different extracellular PSMA epitopes. Moreover, the mAbs 3/E7, 3/F11, and 3/A12 exhibit a similar binding affinity to J591 in C4-2 cells.23 The mAbs 3/E7, 3/F11, and 3/A12 were labelled with 64Cu and evaluated in mice bearing prostate cancer xenografts using microPET. The imaging results reveal high uptake of the mAbs in PSMA-positive C4-2 xenograft at 24 and 48 hours post-administration but not in PSMA-negative DU-145 xenografts.24 The mAb 3/F11 was also evaluated as a radioimmunotherapeutic agent after labelling with 177Lu. The 177Lu labelled 3/F11 exhibits high uptake in C4-2 xenografts with a serum half-life of 7 days. A biodistribution study demonstrated maximum uptake in the tumour at 72 hours post-administration. Furthermore, a single dose of 177Lu-DOTA-3/F11 inhibits tumour growth and improves the survival rate of the mice, indicating the great potential of using 3/F11 as a targeting ligand for prostate cancer therapeutics.25
Instead of immunising animals and using hybridoma technology to discover mAbs, scFv phage display has also been used to identify anti-PSMA antibody fragments. In one study, a scFv phage library was constructed from the hybridoma of the mAb 3/A12, which targets the PSMA extracellular domain.The scFv A5 was identified after several rounds of biopanning on LNCaP cells and purified PSMA, and exhibits high affinity to LNCaP cells with a Kd of 38 nM.26 A recombinant immunotoxin (A5-PE40) containing the toxic domain of Pseudomonas Exotoxin A (PE40) and scFv A5 was then constructed, and showed a very high cytotoxicity in LNCaP cells (IC50=20 pM).26 The same approach was also used to discover another anti-PSMA scFv D7 from the phage library that was constructed from the hybridoma 3/F11. Similarly, the recombinant immunotoxin (D7-PE40) shows high affinity to C4-2 cells and induces cell apoptosis with an IC50 of 140 pM.27 The immunotoxin D7-PE40 significantly reduces the size of established C4-2 xenografts in mice. Meanwhile, the immunotoxin D7-PE40 is well tolerated in mice up to a dose of 20 μg.27
Prostate Cancer-Specific Aptamer
Aptamers are single-stranded oligonucleotides that have high affinity to various molecular moieties. Aptamers can be identified through the systematic evolution of ligands by exponential enrichment (SELEX) from a large pool of random oligonucleotide sequences.28 Using SELEX against the PSMA extracellular domain, Lupold and co-workers29 identified two 40-mer RNA aptamers, termed xPSM-A9 and xPSM-A10, with low nanomolar affinity. The aptamers xPSM-A9 and xPSM-A10 also inhibit the NAALADase activity of PSMA with a Ki of 2.1 nM and 11.2 nM, respectively.29
Due to its high affinity and specificity to PSMA, the aptamer xPSM-A10 was radiolabelled with 64Cu and used as a PET imaging agent for targeted molecular imaging of prostate cancer.30 Moreover, the PSMA aptamers have been widely used for prostate cancer targeted drug delivery systems. For example, the aptamer xPSM-A9 was adopted as a PSMA-specific ligand and conjugated to the surface of a liposome encapsulating doxorubicin.31 The aptamer-conjugated liposomes specifically internalise into PSMA-positive LNCaP cells and subsequently induce cell apoptosis, while neither uptake nor cytotoxicity is observed in PSMA-negative PC-3 cells. A biodistribution study demonstrated high accumulation of doxorubicin in tumour sites at 12 and 24 hours after intravenous administration. Accordingly, an enhanced anti- cancer effect was observed in the animals treated with the A9-conjugated liposome.31
Similarly, PSMA aptamers have been used in small interfering RNA (siRNA) delivery for prostate cancer therapy. Since the discovery of RNA interference, siRNAs have attracted much attention as novel therapeutic agents to treat various diseases. However, lack of efficient delivery systems is still the major hurdle that limits the clinical translation of siRNA. In an exploratory study of siRNA delivery, the A10 aptamer was directly linked to an siRNA to form the aptamer–siRNA chimera to achieve targeted delivery to PSMA-positive prostate cancers.32 The A10 aptamer-siRNA chimera not only shows selective uptake in PSMA-positive cancer cells in vitro but also demonstrates significant inhibition of LNCaP xenograft tumour growth in vivo. This is one of the few pioneering studies showing the in vivo activity of siRNA in animal studies.32
The PSMA aptamer was also explored as a therapeutic agent against advanced prostate cancer because several studies have suggested that PSMA’s enzymatic activity may play important roles in prostate cancer angiogenesis, progression, and metastasis.4,33 In one such study, the PSMA-specific aptamer A9g (truncated form of the aptamer A9) specifically inhibits the migration and invasion of PSMA-positive prostate cancer cells in vitro. A further animal study of the aptamer A9g reveals potent inhibition of metastasis in a xenograft mouse model of metastatic prostate cancer. Data from this study suggest that PSMA inhibitors could be used alone as emerging therapeutic agents for advanced prostate cancer.34
Given the poor stability of RNA molecules under physiological condition, DNA aptamer targeting PSMA was discovered to overcome the poor stability of RNA aptamer. A 48-mer anti-PSMA DNA aptamer (SZTI01) containing a binding site for doxorubicin was identified using SELEX against PSMA. A dimeric form of the aptamer SZTI01 was constructed to form a complex with doxorubicin. The aptamer/ doxorubicin complex is stable at physiological conditions and specifically binds to PSMA-positive C4-2 cells. The complex also induces high cytotoxicity in C4-2 cells but negligible activity in PSMA-null cells, indicating its high selectivity for PSMA-positive cells.35
Prostate Cancer-Specific Peptide
Peptide phage display technology has been widely used for identification of peptide ligands against various molecular targets including cancer-specific antigens. Compared with aptamer and antibody ligands, peptide ligands have several advantages, such as low molecular weight, high permeability, low immunogenicity, ease of synthesis, and flexibility in chemical conjugation.36,37 As a result, a great deal of interest has been focussed on the identification of PSMA-specific peptides. For example, biopanning against a recombinant PSMA extracellular domain was utilised to identify a PSMA-specific peptide that can efficiently inhibit the enzymatic activity of PSMA with an IC50 of 26 μM. A dimeric form of this peptide further increases its binding affinity to PSMA.38 In another study, whole-cell biopanning against PSMA-positive LNCaP cells was conducted to identify an LNCaP-specific peptide (KYL), which can efficiently deliver various cargos to LNCaP cells. Although the KYL peptide shows high and specific binding to LNCaP cells but not to PSMA-negative PC-3 cells, the peptide is not PSMA-specific and its target moiety is unknown.39 We recently designed a combinatorial biopanning strategy against a recombinant PSMA extracellular domain, LNCaP cells, and LNCaP xenografts in nude mice and identified a PSMA-specific peptide (GTI), which shows high affinity to PSMA-positive cells in vitro and in vivo. The Kd values of the GTI peptide to LNCaP and C4-2 cells are 8.22 and 8.91 μM, respectively (unpublished data). One potential problem that may limit the application of peptide ligands is their relative low binding affinity compared with antibodies and aptamers. Most peptides identified from phage display show Kd values in the low micromolar range.40 Therefore, further modification of peptide ligands, such as D-form amino acid modification,41 ligand dimerisation, and tetramerisation38 may be used to further improve their affinity.
Prostate Cancer-Specific Small Molecule
N-acetyl-L-aspartyl-L-glutamate (NAAG) is an endogenous peptide found in the mammalian nervous system. It can be hydrolysed by the N-acetylated α-linked acidic dipeptidase (NAALADase, or NAAG peptidase) with a Km of 540 nM.42 Given the fact that PSMA is highly homologous to NAAG peptidase, several studies have been carried out to design NAAG analogues that can specifically bind to PSMA.43-46
Based on their previous experience in designing NAAG analogues, Pomper et al.43 synthesised two radiolabelled small molecules, [11C]DCMC and [125I]DCIT. Both of these analogues exhibit high accumulation in LNCaP xenograft at 30 minutes post-administration, whereas the uptake in PSMA-negative MCF-7 and PC-3 derived tumours is negligible. Another group synthesised a series of glutamate-urea-X (X is a derivatised lysine) heterodimeric inhibitors with high affinity to PSMA in the low nM range.44 Two of the inhibitors, MIP-1072 and MIP-1095, efficiently inhibit PSMA enzymatic activity with Ki values of 4.6 nM and 0.24 nM, respectively. Moreover, MIP-1072 and MIP-1095 exhibit very high affinity to PSMA (Kd <4 nM).47 Specific binding of 125I labelled MIP-1072 and MIP-1095 was demonstrated in mice bearing LNCaP xenografts.47 These promising data have led to the Phase I clinical trial of these small molecule inhibitors for imaging prostate cancer.48 Clinical studies demonstrate that 123I-MIP-1072 and 123I-MIP-1095 can detect lesions in the prostate gland, soft tissues, and bones at 1–4 hours post-injection. Target-to background ratio is >10:1 at 4 and 24 hours for single photon emission computed tomograph/computed tomography (SPECT/CT).48 Two other PSMA small molecule inhibitors, MIP-1404 and 1405, also show high affinity to LNCaP cells with Kd values of 1.07 nM and 4.35 nM, respectively.46 A clinical study of (99m)Tc-MIP-1404 and 1405 revealed that they can detect soft-tissue prostate cancer lesions including subcentimetre lymph nodes and most bone metastatic lesions.45
It is reported that the active binding site of PSMA is composed of one binding pocket for glutamate-urea-X inhibitors and another lipophilic pocket.49 Eisenhut et al.50 therefore hypothesised that attachment of a hydrophobic moiety to the current glutamate-urea based PSMA inhibitors may enhance their affinity to PSMA. As a result, a novel imaging agent was synthesised by conjugating a hydrophobic side chain, 68Ga chelator-HBED-CC (N,N’-bis[2-hydroxy-5(carboxyethyl)benzyl ethylenediamine-N,N’-diacetic acid), to a glutamate-urea based inhibitor. The new imaging agent exhibits enhanced specificity for PSMA-positive tumour cells in vitro and in vivo. It also demonstrates faster blood and organ clearances and lower liver accumulation in an animal study.50 The [68Ga]PSMA-HBED-CC-PET/CT imaging agent has also been evaluated in prostate cancer patients for the diagnosis, radiotherapy, and management of prostate cancer.51,52 Several other small molecule inhibitors, such as DUPA, DCFBC, and phosphoramidate peptidomimetic have also entered preclinical and clinical studies as imaging agents to detect castration-resistant prostate and metastatic hormone-naïve prostate cancer.53-55
Due to their high affinity and specificity to PSMA, these PSMA small molecule inhibitors have also been investigated for prostate cancer targeted drug delivery. In one study, Low et al.54 conjugated tubulysin, a microtubule inhibitor, to DUPA, a small molecule inhibitor of PSMA with a Kd value of 14 nM. The conjugated drug (DUPA-TubH) exhibits high anti-cancer activity against PSMA-positive prostate cancer cells in vitro and in vivo. Pre-administration of excess PSMA inhibitors abolishes the activity of DUPA-TubH, indicating the high specificity of DUPA-TubH to PSMA. Later, the same group conjugated a series of different chemotherapeutic agents to DUPA and similar activity against PSMA-positive prostate cancer was observed, suggesting flexibility in the use of the PSMA small molecule inhibitor as a targeting ligand for various agents.56 A number of other PSMA-specific small molecules have also been explored as targeting ligands for various therapeutic agents, including radionuclide,57 gold nanoparticles,58 photosensitiser,59 and polymeric microbubbles.60
CONCLUSION
Four types of PSMA-specific ligands and their applications in prostate cancer imaging (Table 1) and therapy (Table 2) are discussed in the review. Although PSMA-specific ligands are mainly used as prostate cancer imaging agents and targeting ligands for drug delivery systems, some of the PSMA inhibitors can also be used alone as anti-cancer therapeutic agents.
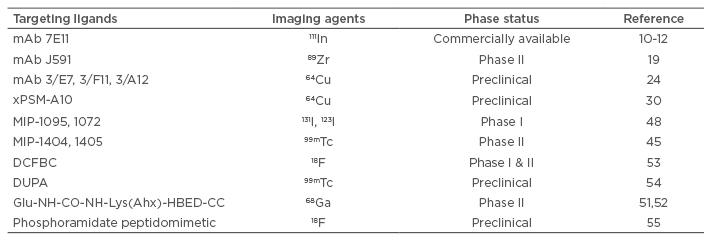
Table 1: Applications of prostate-specific membrane antigen-specific ligands in prostate cancer imaging.
mAb: monoclonal antibody.
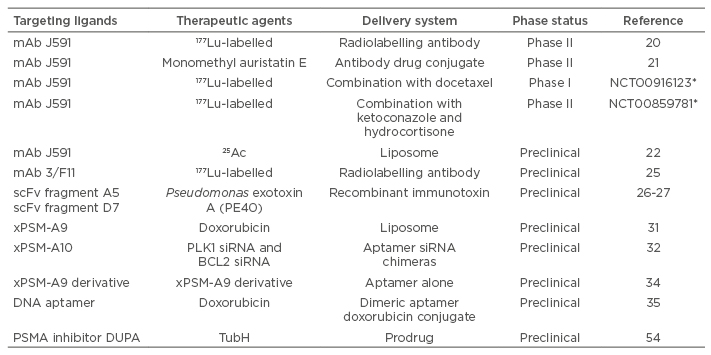
Table 2: Applications of PSMA-specific ligands in prostate cancer therapy.
*ClinicalTrials.gov Identifier (www.clinicaltrials.gov)
mAb: monoclonal antibody; PSMA: prostate-specific membrane antigen; scFv: single chain fragment variable; siRNA: small interfering RNA.
As the first generation PSMA-specific ligand, PSMA mAbs have been widely investigated because of their high affinity and specificity. One of the PSMA mAbs, ProstaScint®, was approved by the FDA for imaging prostate cancer. Several other PSMA mAbs are currently in clinical trials to explore their application as imaging and therapeutic agents. Regardless of its success, the major disadvantages of mAbs are possible immunogenicity, long half-life in the circulation, and low permeability in tumour site due to their large molecular size. As a result, other PSMA ligands with small sizes, such as aptamers, peptides, and small molecule inhibitors have gained much interest in recent years in prostate cancer diagnosis and targeted therapy.
Taken together, a great deal of effort has been devoted to discovering numerous PSMA-specific ligands and exploring their applications in prostate cancer diagnosis and therapy in the past two decades. Although the majority of these applications focus on the imaging of prostate cancer, there is an increasing interest in the use of the ligands in targeted drug delivery systems to increase the efficacy of current prostate cancer therapeutic agents.