Abstract
Bladder cancer treatment remains a challenge in the pharmaceutical field due to the recurrence and progression of the disease, as well as the pronounced side effects associated with the available therapeutic modalities. Although important strategies have been investigated in different clinical trial phases, efficient and well-tolerated treatment approaches need to be developed to improve therapeutic efficacy and the quality of life for bladder cancer patients. This review discusses conventional protocols used in the clinical setting, detailing the use of Bacillus Calmette–Guérin, new immunomodulators, and drug delivery systems. New therapeutic approaches have been investigated with the aim of better therapeutic efficacy with low rates of recurrence and progression of non-muscle invasive bladder cancer and muscle invasive bladder cancer. Therefore, this review highlights the progression of therapy with the use of conventional treatments and the recent progress achieved from the use of innovative strategies, such as nanoparticles for sustained, controlled drug delivery and increased drug uptake by tumour cells.
INTRODUCTION
Overview of Bladder Cancer
Bladder cancer is an important type of cancer worldwide. The European Association of Urology (EAU) considers bladder cancer the eleventh most common cancer diagnosed worldwide.1,2 The American Association for Cancer Research (AACR) estimated that approximately 79,030 new cases of bladder cancer would be diagnosed in the USA in 2017 (60,490 cases in men and 18,540 in women) with approximately 16,870 deaths resulting from bladder cancer (12,240 men and 4,630 women).3
More than 70% of bladder cancer is superficial (i.e., non-muscle invasive bladder cancer [NMIBC]) and classified into three stages: pTis (flat carcinoma in situ), pTa (non-invasive papillary carcinoma), and pT1 (tumour invading the mucosa or submucosa of the bladder wall). Despite the prognosis associated with NMIBC, almost 50% of patients will experience recurrence of their disease within 4 years of their initial diagnosis and 11% will progress to muscle invasive bladder cancer (MIBC).2
Non-Muscle Invasive Bladder Cancer
The primary therapy for high-grade NMIBC is based on surgery in the form of transurethral resection of the bladder tumour followed by intravesical immunotherapy with Bacillus Calmette–Guérin (BCG).1,2 The response induced by BCG reflects induction of a T helper Type 1 response to prevent recurrence and to reduce tumour progression.1,2 However, BCG treatment is associated with therapeutic failure in up to 50% of patients and several side effects occur in up to 90% of patients.2
The treatment of NMIBC remains a challenge in the pharmaceutical field due to the recurrence and progression of the disease, as well as the pronounced side effects that are still associated with the available therapeutic modalities. Although important strategies have been investigated in different preclinical studies and clinical trials, it is crucial to develop innovative drug delivery platforms that allow intravesical drug administration, avoiding the progression of NMIBC to MIBC and thus improving patient quality of life.4
TREATMENT MODALITIES
Immunomodulators
In 2014, a literature review demonstrated that transurethral resection with adjuvant intravesical chemotherapy or immunotherapy is the standard treatment option for NMIBC.5 Interferon (IFN)-α as adjuvant therapy had limited efficacy, but BCG monotherapy was suggested as first-line treatment. However, cancer progression or recurrence was a common outcome, and when treated with radical cystectomy it was associated with significant morbidity and mortality, as well as low quality of life. Unfortunately, chemotherapeutic treatment is not effective after BCG failure.6 There is a lack of efficacious treatment options for patients with NMIBC recurrence or progression after initial BCG treatment.
In 2016, atezolizumab, an immune checkpoint inhibitor (T cell), was approved in metastatic urothelial carcinoma; later, in 2017, nivolumab was also approved.7 Programmed cell death protein 1/programmed death-ligand 1 (PD-1/PD-L1) checkpoint inhibitors have achieved durable clinical responses in a subset of previously treated and treatment-naïve patients with metastatic urothelial carcinoma. The combination of PD-1 and cytotoxic T-lymphocyte antigen 4 has successfully improved response rates, including for bladder cancer.8
BCG-cell wall skeleton (CWS) represents an alternative to the use of live BCG. However, many problems, such as low water solubility and a high molecular weight, have been identified. In order to overcome these obstacles, Nakamura et al.9 developed lipid nanoparticles for BCG loading.
It is known that IFN-α is effective against carcinoma in situ and is also a prophylactic agent after transurethral resection in patients with bladder cancer, which involves the stimulation of the T helper 1 immune response by IFN when associated with BCG.10 Patients who failed BCG treatment were treated with IFN and BCG in lower doses than the conventional protocols for BCG alone.11,12
In a study with 236 patients with frequently recurring Ta or T1 Grade 1–2 NMIBC, treated with mitomycin C instillations followed by monthly BCG, significantly reduced long-term disease recurrence in relation to alternating instillations of BCG and IFN-α2b. Therefore, the combined therapy of BCG with IFN-α offers an appreciable clinical benefit compared with BCG alone.10
Protein Aggregate Magnesium-Ammonium Phospholinoleate-Palmitoleate Anhydride Immunomodulator
The protein aggregate magnesium-ammonium phospholinoleate-palmitoleate anhydride (P-MAPA) comprises a non-linear biopolymer produced by the fungus Aspergillus oryzae during the fermentation process. Studies conducted with P-MAPA in animal models showed this compound to have both anti-tumoural and anti-infectious effects.13-15 The main mechanism by which P-MAPA realises its pharmacological effects comprises the modulation of both immunomodulatory processes and biological responses.
The anti-tumour activity of P-MAPA was evaluated in vivo using a bladder cancer model induced in female Fischer 344 rats.16,17 The anti-tumour assays revealed that P-MAPA increased toll-like receptor (TLR)2, TLR4, and p53 levels. Moreover, it was more effective in treating bladder cancer compared to BCG.16,17 Garcia et al.18 investigated the biological responses due to the association of P-MAPA with flutamide with the aim of potentiating the anti-tumour response compared to BCG, the most commonly used therapy for NMIBC. Data showed that P-MAPA was able to activate the innate immune response via TLR2 and TLR4; this resulted in an increase of IFN signalling compared to BCG, which is consistent with the superior efficacy of P-MAPA in relation to BCG. In this protocol, there was a complete regression in both neoplastic (60% of the animals) and benign tumours (40% of the animals). P-MAPA has demonstrated the ability of activating the innate immune response via TLR2 and TLR4, and also to increase IFN signalling; thus, it may represent a promising alternative for treatment of NMIBC.
P-MAPA intravesical immunotherapy led to distinct activation of the TLR2 and TLR4-mediated innate immune system by increasing the IFN signalling pathway (TRIF-dependent pathway). P-MAPA therapy was found to be more effective in NMIBC treatment than BCG. P-MAPA immunotherapy increased wild-type p53 protein levels with nitric oxide-induced apoptosis and the upregulation of BAX. Therefore, P-MAPA immunotherapy is an important therapeutic strategy for NMIBC, offering a new perspective for the treatment of patients that are refractory or resistant to BCG intravesical therapy.19 Interesting results were observed when using the combination of P-MAPA with cisplatin (CIS) and doxorubicin (DOX).20
DRUG DELIVERY SYSTEMS
Nanoparticulated Delivery Systems
In this important area of nanomaterial, some reviews have been published.21-25
Liposomes
A study evaluated the anti-tumour effect of free BCG-CW and R8-liposome-BCG-CWS using a bladder carcinogenesis model induced in Fischer 344 rats by N-butyl-N-(4-hydroxybutyl)-nitrosamine intravesical administration. The results demonstrated that both free BCG-CW and R8-liposome-BCG-CWS decreased carcinoma incidence and number of tumours compared to untreated animals.26
Duplex dsP21-322 and its chemically modified variants are examples of RNA-based drugs that inhibit cancer cell growth by inducing expression of tumour suppressor p21WAF1/CIP1 (p21). The 20-fluoro-modified derivative (dsP21-322-2’F) was encapsulated into lipid nanoparticles for intravesical delivery. The therapeutic effect of dsP21-322 in an orthotopic model of bladder cancer with lipid nanoparticles, based on formulations used in RNA interference-based therapies consisting of polyethylene-glycol (PEG)-stabilised unilamellar liposomes built with lipid 2,2-dilinoleyl-4-(2-dimethylaminoethyl)-[1,3]-dioxolane, confirmed p21 induction (regression or disappearance of tumour in 40% of the treated mice), cell-cycle arrest, and apoptosis in an in vitro study, following treatment with lipid nanoparticle dsP21-322-2’F.27
BCG-CWS was used to replace live BCG as a bladder cancer drug encapsulated in a liposome. In a functional evaluation, CWS-nanoparticles were efficiently taken up by mouse bladder tumour cell line (MBT-2) cells in vitro and the nanoparticle formation inhibited tumour growth in mice with MBT-2 tumours.28
Another review considered the current status of intravesical liposomes or liposomal-mediated drug delivery for the treatment of interstitial cystitis (also known as painful bladder syndrome) and overactive bladder.29 Flow cytometry analysis demonstrated that the uptake of liposomes at 37°C by cultured human UROtsa cells was via clathrin-mediated endocytosis.30 Application of liposome and micelle-based formulations used in the delivery of platinum-based anti-cancer drugs in clinical trials found an enhancement in the treatment of cancers.31
The introduction of cholesteryl-PEG into the cationic liposomes showed a significant enhancement in cellular uptake by the efficient elimination of the aggregation process. The authors suggested that by incorporating cholesteryl-PEG into cationic liposomes it was possible to achieve both stability in urine and effective cellular uptake.9 The lyso-thermosensitive liposomal DOX (LTLD) (ThermoDox®, Celsion Corporation, Lawrenceville, New Jersey, USA), in combination with loco-regional mild hyperthermia (HT), was used for targeted drug delivery to the porcine bladder wall in vivo. The results demonstrated that DOX accumulation and distribution within the bladder wall was observed at concentrations higher than with free intravenous DOX by mild bladder HT combined with systemic delivery of LTLD.32
Generally, in the treatment of bladder cancer it is desirable to preserve the bladder rather than perform a partial or full cystectomy. Bladder cancer patients who express high levels of polo-like kinase-1 (PLK-1) are known to have a poor prognosis. In this direction, intravesical PLK-1 small interfering RNA (siRNA) was studied. siRNA/cationic liposomes (the cationic liposome used was DOTMA) suppressed the expression of endogenous PLK-1 in a time and dose-dependent manner within bladder cancer cells.33 In an in vivo study using an orthotopic mouse model and the LUC-labelled bladder cancer cell line (UM-UC-3 LUC), PLK-1 siRNA was transfected into the cells, reducing PLK-1 expression and inhibiting the growth of BC.34 The summary of these results is presented in Table 1.
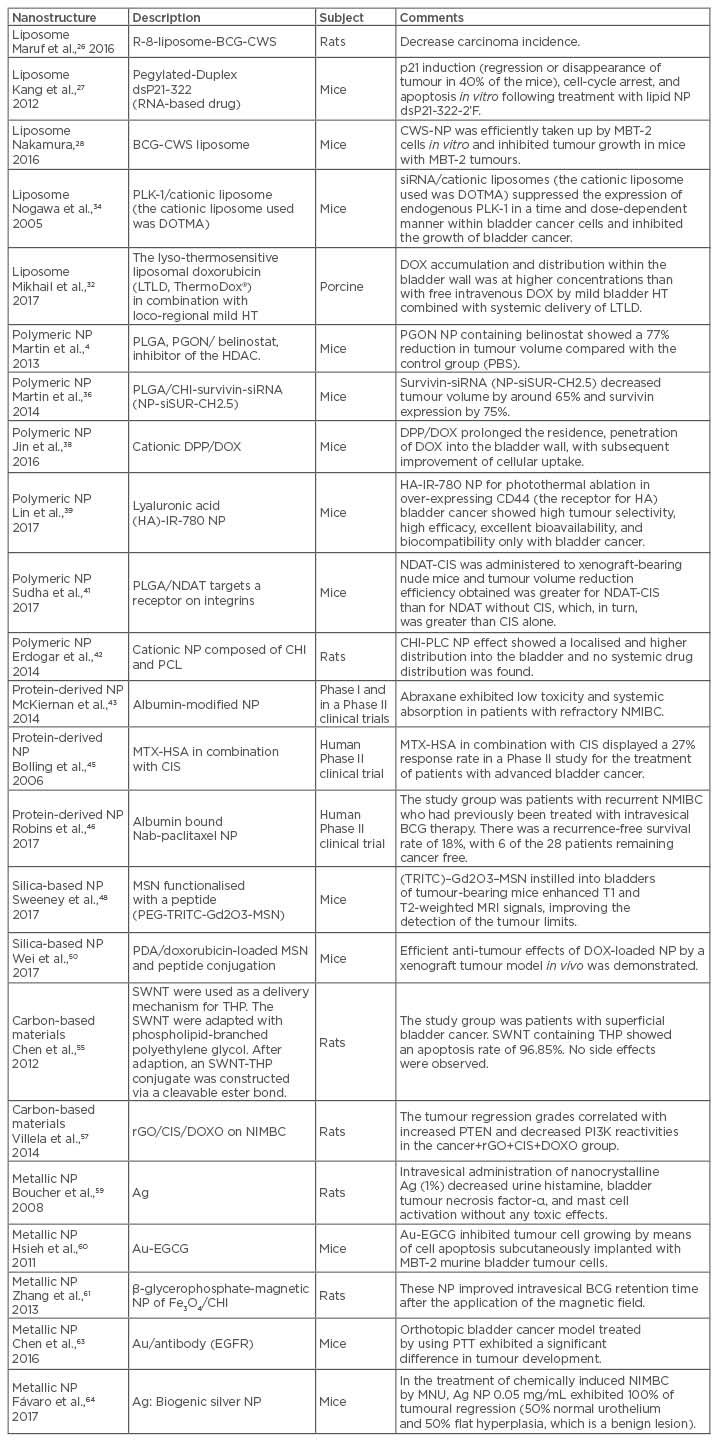
Table 1: Properties of nanoparticles and their effects on in vivo experiments.
Ag: silver; Au: gold; BCG: Bacillus Calmette–Guérin; BCG-CWS: BCG cell wall skeleton; CD: cluster of differentiation; CIS: cisplatin; CHI: chitosan; CWS-NP: cell wall skeleton-nanoparticle; DOTMA: N-(1-[2,3-dioleyloxy]propyl)-n,n,n-trimethylammonium chloride; DOX: doxorubicin; DPP: 1,2-dioleoyl-3-trimethylammonium propane/methoxypoly (ethyleneglycol); EGCG: (-)-epigallocatechin-3-gallate; EGFR: epidermal growth factor receptor; Fe: iron; HA: hyaluronan; HDAC: histone deacetylase; HT: hyperthermia; MBT-2: mouse bladder tumour cell line; MTX-HAS: methotrexate-albumin; MSN: mesoporous silica nanoparticles; Nab: nanoparticle albumin bound; NDAT: nano-diamino-tetrac (tetraiodothyroacetic acid); NDAT-CIS: nano-diamino-tetrac (tetraiodothyroacetic acid-cisplatin); NIMBC: non-muscle invasive bladder cancer; NP: nanoparticle; O: oxygen; PBS: painful bladder syndrome; PDA: polydopamine; PGON: poly(guanidinium oxanorbornene); PI3K: phosphatidylinositide 3-kinase; PLC: poly-ε-caprolactone; PLGA: poly(lactic-co-glycolic acid); PLK: polo-like kinase; PTEN: phosphatidylinositol-3,4,5-trisphosphate 3-phosphatase; PTT: photothermal therapy; rGO: reduced graphene oxide; SWNT: single-walled carbon nanotubes; THP: tetrahydropyranyl-adriamycin; TRITC: tetramethylrhodamine isothiocyanate.
Polymeric Nanoparticles
Poly-ε-caprolactone (PCL) derivative, PCL-b-P(PMA-click-MSA-co-PEGMA)-loading superparamagnetic iron oxide nanoparticles (SPION), and the pendant dicarboxylic groups in the hydrophilic shell were used to co-ordinate CIS. The new CIS-conjugated polymeric nanoparticles nanostructure (Pt–Fe–PN) is superparamagnetic and mucoadhesive. The authors suggested that Pt–Fe–PN is potentially a promising CIS delivery vehicle in combination with SPION-inducing HT for bladder cancer therapy.35
Martin et al.4 developed poly(lactic-co-glycolic acid) (PLGA) nanoparticles functionalised with polyguanidinium oxanorbornene (PGON), a synthetic polymer able to mimic cell penetrating peptides that are not toxic to mammalian cells. Nanoparticles were developed for delivery of belinostat, an inhibitor of the histone deacetylase. In vivo murine experiments with xenograft tumours were performed for the evaluation of anti-tumoural responses. The results revealed that tumours treated with PGON nanoparticles containing belinostat were 77% smaller in volume compared to those of the control group (painful bladder syndrome).4
In another murine study, Martin et al.36 functionalised the surface of PLGA nanoparticles with the mucoadhesive and positively charged polymer chitosan (CHI) in an attempt to improve the drug retention over the urothelium surface and to enhance drug absorption through this tissue. Chitosan-derived nanoparticles containing survivin-siRNA (NP-siSUR-CH2.5) decreased tumour volume by around 65% and survivin expression by 75%.
Recently, the anti-tumour activities and the mechanisms of hyaluronan (HA)/CHI nanoparticles-aggregated heteronemin (HET) were studied. Pure HET increased early and total apoptosis and JC-1 monomer fluorescence; however, HA/CHI nanoparticles-aggregated HET induced higher apoptosis and JC-1 monomer rates than pure HET. These data strongly indicated that HA/CHI nanoparticle-aggregated HET would be a potential approach for the treatment of in vivo urothelial cancer.37
Cationic 1,2-dioleoyl-3-trimethylammonium propane/methoxypoly (ethyleneglycol) nanoparticles encapsulating DOX for intravesical therapy of bladder cancer were tested in a murine model. The treatment prolonged the residence and penetration of DOX in the bladder wall, with subsequent improvement in cellular uptake.38 Self-assembled tumour-targeting hyaluronic acid-IR-780 nanoparticles for the photothermal ablation of cells over-expressing the HA receptor CD44 in bladder cancer showed high tumour selectivity, high efficacy, excellent bioavailability, and biocompatibility only for bladder cancer.39
In 2017, Canada-based biotechnology firm Sitka Biopharma Inc., Vancouver, Canada, with Cancer Research UK and Cancer Research Technology, London, UK initiated a Phase I clinical trial of STK-01 (hyperbranched polyglycerol docetaxel) for treatment of patients with NMIBC.40 It is known that nano-diamino-tetrac(tetraiodothyroacetic acid) (NDAT) targets a receptor on integrin αvβ3; αvβ3 is expressed by cancer cells and dividing endothelial cells. The NDAT was covalently bound to a PLGA nanoparticle that is able to encapsulate CIS. CIS-loaded NDAT was administered to xenograft-bearing nude mice. The tumour volume reduction efficiency obtained by CIS-loaded NDAT was greater than NDAT without CIS, which, in turn, was greater than CIS alone.41 Erdogar et al.42 developed cationic nanoparticles composed of CHI and PCL for intravesical administration of mitomycin C inrats, avoiding toxicity. Histopathological analysis of the CS-PLC nanoparticles effect showed a localised and higher distribution into the bladder and no systemic drug distribution (Table 1).
Protein-Derived Nanoparticles
Paclitaxel, a poor water-soluble drug, was encapsulated in albumin-modified nanoparticles in order to increase drug water solubility and to improve drug delivery to target cells (Abraxane™, Celgene Corporation, Summit, New Jersey, USA). In Phase I and Phase II clinical trials, the nanoparticles exhibited low toxicity and systemic absorption in patients with refractory NMIBC (T1, Ta, or Tis stage).43 ABI-009 (rapamycin) proved to be effective against colon and breast tumours in xenograft studies. In addition, ABI-009 exhibited low toxicology and good efficacy and has reached a combined Phase I and Phase II study for the treatment of NMIBC.44
Methotrexate-albumin was used in combination with CIS in the treatment of patients with bladder cancer in a Phase II study with a 27% response rate. Methotrexate-albumin has not been taken further for clinical studies.45
A long-term follow-up of a Phase II trial of intravesical nanoparticle albumin-bound paclitaxel for patients with recurrent NMIBC after previous intravesical BCG therapy demonstrated that 6 of the 28 patients remained cancer free, with a recurrence-free survival rate of 18%. The authors suggested that nanoparticle albumin-bound paclitaxel is a reasonable treatment option in this high-risk population.46
Silica-Based Nanoparticles
Silica-based nanoparticles modified with thiol groups provided stronger interactions with the bladder urothelium in relation to nanoparticles modified with hydroxyl and amino groups. Nanoparticles functionalised with thiol loaded with DOX demonstrated that thiol-functionalised mesoporous silica nanoparticles (MSN) are promising as a mucoadhesive and sustained drug delivery system in acidic conditions.47
Sweeney et al.48 described the application of novel MSN functionalised with a peptide that specifically binds to bladder cancer cells, PEG-tetramethylrhodamine isothiocyanate-Gd2O3-MSN, thus improving specificity. In an in vivo model, MSN instilled into bladders of tumour-bearing mice enhanced T1 and T2-weighted magnetic resonance imaging signals, improving the detection of the tumour limits. These facts support the idea that this targeted nanomaterial presents new options for premature detection and possible therapeutic intervention.
Thiolated silica nanoparticles retention on porcine bladder mucosa in vitro, following irrigation with artificial urine solution, was reported. After irrigation with artificial urine solution, the thiolated nanoparticles demonstrate significantly greater retention (Wash Out [WO]50 value: up to 36 mL) compared to non-mucoadhesive dextran (WO50: 7 mL) but have weaker mucoadhesive properties than CHI (WO50: 89 mL).49
A polydopamine-based surface modification method was used to prepare a targeted DOX-loaded MSN and peptide conjugation, which exhibited release kinetic profiles similar to DOX. Efficient anti-tumour effects of DOX-loaded nanoparticles by a mouse xenograft tumour model in vivo were demonstrated.50 These data are discussed in Table 1.
Carbon-Based Materials
Many forms of carbon-based material, such as carbon nanotubes, graphenes, graphene oxide (GO), and carbon nanofibres, are useful in biological applications.51,52 As for GO, the addition of specific groups to the carbon nanotubes surface may improve the solubility and decrease toxicity of these nanostructures.53
A Pt(IV)-based single-wall carbon nanotube (SWNT)-mediated pro-drug led to intracellular concentrations of Pt4+ six-fold higher than when the testicular carcinoma cell line (NTera-2) was treated with the free drug. In addition, the cytotoxicity induced by CNT-loaded carboplatin was significantly higher than that provided by the free drug.21,54
Pirarubicin (tetrahydropyranyl-adriamycin [THP]), associated with SWNT through phospholipid-branched polyethylene glycol, showed no side effects with SWNT alone or with SWNT-THP treatments on rat bladder cancer. In vivo studies with rat bladder cancer as in situ models constructed by N-methyl-N-nitrosourea intravesical installation showed apoptosis rates of 96.85% for SWNT-THP. No side effects were observed in the SWNT-THP group.55
In an animal model for NMIBC, in which 100% of animals from the cancer group presented with carcinoma with invasion of the lamina propria (pT1) and squamous metaplasia, the effects of reduced GO (rGO) was studied. The best histological recovery was found in the rGO/CIS/DOX group, showing 40% had flat hyperplasia, 40% had low-Grade intraurothelial neoplasia, and 20% had pTis. Taken together, it was suggested that GO had potential as a carrier system of chemotherapeutic agents, such as DOX, and as nanoformulation for bladder cancer treatment (Table 1).51,56,57 A binary detection system for the simultaneous detection of miRNA and telomerase using the concept of integration of signal amplification by nicking with the photo-quenching ability of GO has been developed. The most important result in this report was that this approach can differentiate MIBC from NMIBC, which will benefit clinical decision-making for personal treatment.58
Metallic Nanoparticles
Intravesical administration of nanocrystalline silver (1%) in rats decreased urine histamine, bladder tumour necrosis factor-α, and mast cell activation without any toxic effect, and these activities could be useful for interstitial cystitis.59 (-)-Epigallocatechin-3-gallate was physically attached onto the surface of gold nanoparticles (GNP), and the anticancer activity of the (-)-epigallocatechin-3-gallate-adsorbed GNP inhibited tumour cell growth by means of cell apoptosis in C3H/HeN mice subcutaneously implanted with murine bladder tumour-2 cells.60
A hydrogel formulation (CHI and β-glycerophosphate-magnetic nanoparticles of Fe3O4) improved intravesical BCG retention time after the application of the magnetic field in rats.61 Castiglioni et al.,62 using both bare and polyvinylpyrrolidone-coated silver nanoparticles on T24 bladder cells, showed that the two types of silver nanoparticles promoted morphological changes and cytoskeletal disorganisation. Previous studies have reported the application of modified GNP in bladder cancer. These modified nanoparticles include gum arabic-coated radioactive, hyaluronic acid functionalised fluorescent, epigallocatechin-3-gallate, and antibody-coated silica nanoshells. To target bladder cancer, epidermal growth factor receptor, mucin 7, and cytokeratin 20 are commonly used.22
Photothermal therapy (green laser-532 nm) for targeting superficial bladder cancers using a green light laser in conjunction with GNP conjugated to antibody fragments (anti-epidermal growth factor receptor) resulted in the production of a thermal energy to kill urothelial carcinomas at very low energy levels, in both in vitro and in vivo mice models. In an orthotopic bladder cancer model, animals treated by using photothermal therapy exhibited significant differences in tumour development.63
Biogenically synthesised silver nanoparticles suspensions were intravesically injected in 20 female mice (C57BL/6),64 with NMIBC induced by administration of N-methyl-N-nitrosourea.19 The urinary tract in the control animals did not show microscopically alterations. In contrast the N-methyl-N-nitrosourea group exhibited drastic histopathological alterations, such as urothelial carcinoma with invasion in the lamina propria and urothelial papillary carcinoma. For animals treated with N-methyl-N-nitrosourea and silver nanoparticles 0.05 mg/mL, the authors reported 100% tumour regression (50% normal urothelium and 50% flat hyperplasia, which is the beginning of the lesion) (Table 1).64
CONCLUSION
Nanotherapies are a promising approach for bladder cancer treatment due to their excellent results. The combination of nanomaterials with chemotherapeutic agents is able to provide a sustained and controlled drug delivery directly to the target site of application, increasing drug uptake by tumour cells and decreasing the side effects. This disruptive innovation must be seriously considered with regards to design safety, as this will permit important developments; however, more studies are required in this field.