Abstract
The use of musculoskeletal ultrasound is expanding in many medical disciplines, and simulation trainers have been successfully employed to help practitioners learn various ultrasound techniques. While there are fewer commercial trainers in musculoskeletal ultrasound than other ultrasound modalities, the ones that do exist can be prohibitively expensive. Several less expensive phantom trainers have been described in the literature, including those made of ballistic gelatine. The authors present a three-dimensional printed knee phantom that was overlaid with ballistic gelatine as a viable option for training.
INTRODUCTION
The use of ultrasonography has been increasing across many specialties, including rheumatology.1,2 Interest in ultrasonography by clinicians, termed point-of-care ultrasound, has risen dramatically; this is attributable to evidence for improved patient safety, more rapid diagnostic assessments, and assistance with procedural guidance.3 Among point-of-care ultrasound modalities, musculoskeletal ultrasound (MSUS) has been of particular interest to rheumatologists. Although MSUS is used by rheumatologists in the USA less frequently than in Europe, standardised training programmes have been developed to narrow the utilisation gap.4,5
In a recent survey of MSUS instruction in adult rheumatology fellowship programmes in the USA, 80% of programmes that offered MSUS training included ultrasound-guided injections and procedures as a programme topic.5 The knee is the most common joint that may require synovial fluid aspiration in rheumatology practice, regardless of the use of ultrasound-guidance.6 Across specialties, knee injections and aspirations have typically been performed using anatomic landmarks to determine needle placement.
Effusions of the knee joint have various causes. With the knee in extension, fluid accumulates in the suprapatellar pouch. The collection of fluid in the suprapatellar pouch allows for aspiration of joint fluid, and arthrocentesis is typically performed with a medial or lateral approach.7 When fluid collections are smaller, careful redirection of the needle may be required to access the suprapatellar pouch. Redirection of the needle can increase patient discomfort and chance of trauma to the femoral and patellar cartilage, as well as prolong the duration of the procedure.
Ultrasound guidance of intra-articular knee aspiration and injection increases accuracy compared to the landmark-based approaches.8,9 Overall, an ultrasound-guided aspiration is similar to the landmark-based approach, although techniques vary between clinicians. Using a linear ultrasound probe, the suprapatellar pouch with effusion is identified in long-axis to the quadriceps tendon. The probe is then turned 90 degrees to identify the effusion in short-axis to the quadriceps tendon. A needle is inserted and visualised entering the effusion, and the contents are aspirated. Additionally, the injection of corticosteroids, or other agents, can be performed once the needle is visualised in the suprapatellar pouch.
Ultrasound training programmes have been developed across medical specialties and in many medical schools, and simulation training phantoms have been used to supplement education.10-12 Rather than attempt a previously unperformed procedure on a patient, a trainee can practice procedures on a procedural phantom prior to attempts on patients. Fidelity of the model is paramount. The use of simulation phantoms improves competency,13 but the cost of such phantoms may be unaffordable for many training programmes. Blue Phantom, a commercial simulation training company, has developed a knee ultrasound training model at the cost of $3,799.14
As a result of the high cost of commercially available phantoms, many clinical ultrasonographers have developed low-cost, homemade phantoms.15,16 Various materials have been used for these phantoms, including gelatine, agar, and food substances. Ballistic gelatine has recently been described as a reusable material well suited for procedural training.17 For anatomical guidance, pre-moulded models have been purchased and embedded in ballistic gelatine.18
A functional knee joint model can cost from $30 to $100.19,20 While clearly less expensive than the commercial knee ultrasound model, this cost could still be excessive to some. In addition, the presence of metal screws may create undesirable artefacts. Three-dimensional (3D) printing has been introduced as an inexpensive way to create prototypes of numerous objects and has been used in the printing of anatomical models.21 To the best of the authors’ knowledge, no literature exists on the use of 3D-printing to create an ultrasound procedural phantom of a human joint.
This article proposes a 3D-printed anatomical knee model embedded in ballistic gelatine as a low-cost ultrasound phantom for procedural guidance of suprapatellar needle placement.
MATERIALS AND METHODS
Knee models were created using open-source digital image manipulation software, a 3D printer, and common household materials.
- The initial digital CT model of the knee bones was acquired from the open-source platform Thingiverse22 and edited using Blender.
- Cruciate ligament models were acquired from a Digital Imaging and Communications in Medicine (DICOM) MRI dataset.23 The ligaments were selected from the MRI data using InVesalius (CTI 2017). A 3D model was created from the DICOM slices using InVesalius. The 3D rough model was exported to Blender for cleaning and preparation for use with the 3D printer. After cleaning in Blender, the finalised digital file was exported as a .STL file to Cura (Ultimaker 2016), which created instructions for the 3D printer.
- The 3D printer (LULZBOT TAZ6, Aleph Objects 2016) used the instructions from Cura to render the model in plastic.
- The bony model was affixed with the ligament models using heated metal pins to melt the two structures together into a functional knee joint with accurate placement of cruciate ligaments.
- The printed knee was fitted with menisci and collateral ligaments, which were made from hot glue.
- The pre-femoral, quadriceps, and Hoffa’s fat pads were moulded using modelling putty, and the suprapatellar effusion was created using negative space between the putty. Nitrile glove strips were secured over the putty to create the anterior portions of the quadriceps and patellar tendons.
- Ballistic gelatine was mixed in a 10% consistency as previously described.17
200 g of Vyse professional grade ballistic gelatine (Gelatin Innovations Inc., Schiller Park, Illinois, USA) was dissolved in 1.8 L of water to create a 2.0 L mixture.
A heated magnetic stirrer with stir bar was used to heat the ballistic gelatine mixture to a boil.
Four drops of defoamer (Gelatin Innovations Inc.) was added to prevent foaming.
Congealing was prevented by turning off the heating element of the stirrer while the stir bar continued to stir. Red food colouring was added.
- The 3D-printed knee model was embedded in liquefied ballistic gelatine within a cylindrical food storage container, and an ice bath was used to surround the container to prevent melting of modelling putty. Once cooled and solidified, the phantom was removed from the container (Figure 1).
Ultrasound images of the phantom were acquired with a GE Venue 40 ultrasound machine and compared to a human suprapatellar effusion (Figures 2 and 3).
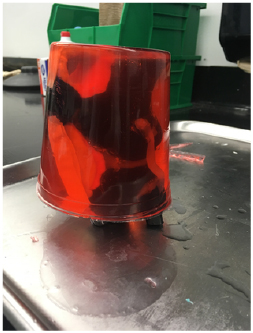
Figure 1: Completed phantom embedded in ballistic gelatine.
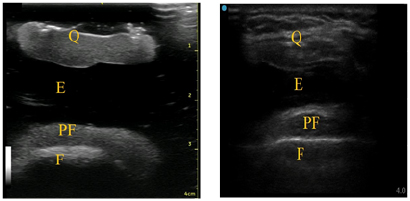
Figure 2: A) Suprapatellar short-axis view of knee phantom; B) human suprapatellar effusion ultrasound anatomy in short-axis.
E: effusion; F: femur; PF: pre-femoral fat pad; Q: quadriceps tendon, quadriceps fat pad.
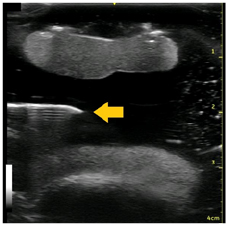
Figure 3: Knee phantom in suprapatellar short-axis view with effusion.
Arrow: needle placement.
RESULTS
The sonographic appearance of the 3D-printed knee phantom was comparable to those observed from a human with suprapatellar effusion. The ballistic gelatine soft tissue component worked well for repeated needle insertions and was easy to remove, melt, and re-cast as desired. Needle placement into the suprapatellar pouch was performed with good technique correlation to human anatomy. The approximate cost of the materials to create the phantom was $50.
DISCUSSION
The major strengths of this knee phantom were its fidelity to a human knee effusion, low cost, and reusability. For a procedural phantom to be effective in improving the competency of trainees, it must be highly comparable to its source. By using a digital knee joint model created from a CT image, the size and contours of the bony components were precise, and there were no undesirable artefacts, such as screws, found on pre-made knee models.
Ultrasound imaging of suprapatellar pouch in short-axis showed soft-tissue landmarks comparable to those in a human knee with effusion. Finally, the anechoic effusion of the phantom was nearly identical to that seen in a human knee effusion. The technique required to visualise needle placement into the effusion was also comparable to that used on patients.
When compared to the $3,799 cost of the Blue Phantom commercial knee ultrasound training model, the $50 materials cost of the 3D-printed phantom was significantly less expensive. Many universities have 3D printers available for use, which would eliminate the need to purchase a 3D printer. All software used was open-source. The reusability of the 3D-printed knee phantom also allowed for further cost savings. Because the only area of the phantom that could be punctured was the ballistic gelatine outer component, the 3D-printed knee bones and soft-tissues remained intact. The outer ballistic gelatine could be removed, reheated, and reapplied without the need to purchase additional equipment.
Limitations of the Three-Dimensional-printed knee phantom
The 3D-printed knee phantom was not without limitations, however. When compared with the commercial knee phantom, there was no outer covering to replicate skin and the musculoskeletal landmarks used for palpation. Ideally, a phantom would provide the ability for both landmark-based and ultrasound-guided aspiration and injection. The cylindrical design of the 3D-printed phantom was created by the food storage container to allow for embedding of the knee within the ballistic gelatine, which limited its ability to be used for landmark-based procedures.
An additional limitation was the transparency of the outer gelatine layer. The ballistic gelatine used in the outer layer was dyed red, but the bones and soft tissues remained visible despite the dye. To ensure a trainee is unable to visualise the needle without the ultrasound, a darker dye is needed to force reliance solely on ultrasound visualisation of the effusion.
Finally, the effusion on the phantom was created using negative space that was filled with ballistic gelatine during the embedding process. While the anechoic gelatine was visually consistent with a human suprapatellar effusion, there was no fluid to aspirate. Creation of a reusable, fluid-filled pouch would have been technically challenging and increased both the complexity and the cost of the phantom. Commercial phantoms typically have fluid-filled compartments that can provide the haptic feedback of accessing the suprapatellar effusion.
CONCLUSION
Ultrasound phantoms have high value as learning tools for both novices and more experienced ultrasonographers because of their versatility. Since the advent of 3D-printing and the ability to create custom phantoms derived from scans of patient anatomy, the opportunity for the clinician to practice a procedure prior to performing it on the patient exists and provides the potential for a lower degree of errors with greater patient safety. This article demonstrated a versatile and fairly simple method for producing a low-cost, reusable knee phantom. This method can be tailored and modified to most, if not all, joints of the human body, and the resultant designs can be made available online and shared globally in an open-source manner. Additionally, 3D-printed ballistic gelatine phantoms for ultrasound-guided joint procedures could be used to more quickly increase the competency of trainees.