Abstract
Interstitial lung disease (ILD) is a common and serious complication of systemic autoimmune rheumatic diseases (SARD). The prevalence and prognosis of ILD vary depending on the type and severity of the underlying SARD. Recently, two drugs have been approved by the U.S. Food and Drug Administration (FDA) for the treatment of ILD in SARD, and the American College of Rheumatology (ACR) has issued guidelines for the screening and monitoring of these patients. Therefore, it is essential to detect and manage ILD in SARDs as early as possible. High-resolution CT of the chest is the current gold standard test for diagnosis of ILD, yet, it is not uniformly performed as an initial diagnostic test. This review discusses the latest advances in imaging techniques for the diagnosis, assessment, and follow-up of SARD-ILDs.
Key Points
1. High-resolution CT scan of the chest is widely recognised as a screening imaging tool for interstitial lung disease (ILD), and is more available.2. There is a role for innovatively repurposing existent imaging modalities, such as ultrasound, MRI, or PET-CT, to better characterise ILD and assess for progression.
3. Incorporation of artificial intelligence and machine learning models can serve as an important tool to consistently quantify and characterise ILD by radiologists.
INTRODUCTION
Interstitial lung disease (ILD) is a relatively frequent manifestation of systemic autoimmune rheumatic disorders (SARD), including systemic sclerosis (SSc), rheumatoid arthritis (RA), idiopathic inflammatory myopathies (IIM), primary Sjögren’s syndrome (pSS), anti-neutrophil cytoplasmic antibody-associated vasculitis, and rarely, systemic lupus erythematosus (SLE). ILD in SARD (SARD-ILD) is considered to be a serious end-organ complication, and has a significant impact on morbidity, work productivity, quality of life, and mortality.1 The natural history, in terms of onset and progression, is variable between SARDs, and even among the same patients with SARDs. The ILD manifestation of a given SARD may precede, coincide, or succeed the extrapulmonary manifestation. The screening for ILD may vary between SARDs, depending on risk factors. When suspected, high resolution CT (HRCT) of the chest remains the centre piece to confirm the diagnosis, besides obtaining pulmonary function tests (PFT), and testing the exercise capacity with hall walk testing.2 This review focuses on key factors in screening, diagnosis, and imaging innovations of SARD-ILD.
CLINICAL RELEVANCE OF THIS REVIEW
Therapeutic options, although limited, continue to grow in the management of patients with SARD-ILD. Alongside, there are major advancement in the imaging techniques for earlier detection of ILD. This review describes key and recent developments in imaging to screen and monitor SARD-ILD. Through this review, the authors project what the future may hold in the application of the imaging innovation in different phases of ILD care (Figure 1).
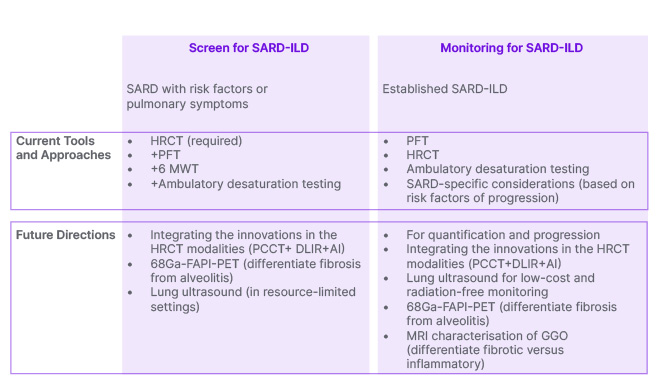
Figure 1: Screening and monitoring for interstitial lung disease in patients with systemic autoimmune rheumatic diseases: current tools and future directions.
AI: artificial intelligence; DLIR: deep learning image reconstruction; GGO: ground-glass opacity; HRCT: high-resolution CT; PCCT: photon-counting CT; PFT: pulmonary function test; SARD-ILD: systemic autoimmune rheumatic disease-related interstitial lung disease; 6MWT: 6-minute walk test; 68Ga-FAPI-PET: gallium 68–labelled fibroblast activation protein inhibitor positron emission tomography.
SCREENING AND DIAGNOSIS FOR INTERSTITIAL LUNG DISEASE IN SYSTEMIC AUTOIMMUNE RHEUMATIC DISEASES
When Should Screening Be Considered?
Often, ILD develops after a diagnosis of SARD. However, subclinical ILD in SARDs like SSc, confirmed by HRCT, can precede, or appear concomitantly.3 The prevalence estimates vary by study, and by type of SARD. Most studies report higher prevalence in SSc, mixed connective tissue disease, RA, and IIM (30–60%), with lower prevalence in pSS and SLE.4-11 ILD is one of the leading causes of death in SSc and RA,12-15 and because of the high prevalence of ILD in SSc, all patients with SSc should be screened at the time of their diagnosis, even if they are asymptomatic.16 Male African-American patients, patients positive for the anti-Scl-70 antibody, with diffuse cutaneous SSc subset, reflux/dysphagia symptoms, and cardiac involvement, are at high risk for developing progressive ILD.17
In patients with RA, ILD is the second leading cause of death after cardiovascular complications. The timing of ILD development is unclear. In one study, approximately one-third of patients developed ILD in the first year of RA diagnosis, and under one-fifth of them between 1–5 years of RA diagnosis.6 A few known risk factors for the development of ILD are old age, male sex, smoking, positive rheumatoid factor or anti-citrullinated peptide antibodies, and high RA disease activity.6,18 Besides these risk factors, screening for ILD should be considered in patients with RA showing respiratory symptoms (persistent cough or shortness of breath) and Velcro crackles on physical exam.18,19
In patients with IIM, ILD prevalence is variable, and has been reported to be upwards of 70% in longitudinal follow-up.20 The presence of anti-synthetase antibodies and anti-MDA5 antibodies is considered high risk, and should necessitate screening irrespective of pulmonary symptoms.21,22 In other SARDs, namely pSS, anti-neutrophil cytoplasmic antibody-associated vasculitis, and SLE, screening for ILD is based upon patient symptomatology.
Current Screening and Diagnostic Modalities
Screening for ILD should involve a comprehensive clinical history in order to identify symptoms, risk factors, and potential exposures. A detailed physical examination involves lung auscultation for crackles, clubbing, and any physical signs suggesting underlying autoimmune diseases. The gold standard for screening ILD is HRCT.23 Other essential evaluations include pulmonary function testing (including diffusing capacity for carbon monoxide [DLCO], PFT), assessment of exercise capacity (such as the 6-minute walk distance), and ambulatory desaturation testing.
Pulmonary function testing involves measurement of forced expiratory volume, forced vital capacity, total lung capacity, residual volume, and DLCO. PFT is a vital tool in screening patients with SARD in conjunction with HRCT. While 6-minute walk distance and ambulatory desaturation testing are needed as a part of comprehensive diagnostic evaluation, they should not be used in isolation for screening purposes.24
HRCT is optimised to enhance spatial resolution by using reconstruction (recon) algorithms to enhance edge detail, and by using thin (1–2 mm) slice recons to better detect and more accurately characterise subtle abnormalities of the lung parenchyma. In addition to standard supine inspiratory scanning, an HRCT protocol often includes additional prone imaging (to help differentiate between atelectasis and fibrosis), and expiratory imaging (to detect air trapping). HRCT protocols are usually obtained without intravenous contrast, which does not add to pulmonary parenchymal evaluation.
According to the proposed 2023 guidelines by the American College of Rheumatology (ACR), for people with SARDs at increased risk of developing ILD, it is recommended against screening with surgical lung biopsy or transbronchial biopsy.24 In certain instances, patients may require a biopsy for a more definitive diagnosis. In such situations, less invasive options could be explored, like transbronchial biopsy or cryobiopsy. More invasive surgical lung biopsy should only be considered in select situations for diagnostic purposes. The decision to undergo a biopsy should be taken after a collaborative consensus driven by multidisciplinary discussion.
Once Diagnosed, What Is the Role Of Imaging in Interstitial Lung Disease Monitoring?
Studies in patients with SSc-ILD provide the most robust guidance on monitoring in patients with subclinical or clinical SARD-ILD. The ACR 2023 ILD guidelines have provided guidance on monitoring, and vary by the SARD.24 Periodic assessment of symptoms, PFTs, and ambulatory desaturation (every 3–12 months) is considered essential in monitoring. Repeating an HRCT should be considered when clinically indicated. In patients with either IIM-ILD or SSc-ILD, PFTs are recommended every 3–6 months for the first year, then less frequently once stable. In patients with RA-ILD, pSS-ILD, and mixed connective tissue disease ILD, PFTs are recommended every 3–12 months for the first year, then less frequently once stable.
INNOVATIONS IN IMAGING FOR SCREENING AND MONITORING OF INTERSTITIAL LUNG DISEASE IN SYSTEMIC AUTOIMMUNE RHEUMATIC DISEASES
CT: Technical Innovations
HRCT has been an important modality for imaging-based detection, characterisation, and quantification of ILD for nearly 5 decades.25 Since its arrival in the 1980s, incremental advances in CT technology have increased the role of HRCT in the detection, diagnosis, and management of ILD. For example, the shift from second-generation CT towards third-generation helical multidetector CT dropped the scanning time, making single-breath-hold chest CT a reality. This also allowed for a volumetric (as opposed to axial ‘step-and-shoot’) scanning mode. The ensuing benefits include improved image quality, decreased radiation exposure, and obviation of the need to choose between total lung coverage versus high spatial resolution reconstructions. All chest CT scans could now be high-resolution.26
Photon-Counting CT Detector Technology
The maturation of photon-counting CT (PCCT) detector technology is a significant technological shift in the field of HRCT. The ILD-relevant improvements that PCCT brings to the application of HRCT include improved spatial resolution (through new detector design), and decreased image noise (through inherent detector spectral sensitivity).27 PCCT scanners are now a reality in clinical practice, with the first U.S. Food and Drug Administration (FDA)-approved PCCT system made available in 2021.8 Pre-PCCT scanners have a detector size of 0.500–0.625 mm, with one notable exception,28 which limits the spatial resolution of standard CT technology. With PCCT, the discreet detector element, which must be optically isolated from surrounding elements, is replaced by a semiconductor detector element with associated pixellated anodes.27 The size of the anode determines the spatial resolution of the system; these anodes can be made smaller more easily than the scintillation crystals used in non-PCCT. PCCT can reconstruct slices as thin as 0.2 mm, resulting in significant improvements in spatial resolution, which is a game changer for better characterising ILD.
A variety of CT findings characterise ILD, including reticulation, traction bronchiectasis/bronchiolectasis, consolidation, ground glass, nodules, airway thickening, air-trapping, peribronchovascular versus peripheral distribution, etc.29 All these findings are easier to accurately detect and characterise with better spatial resolution. In short, PCCT results in much better HRCT image quality, which allows radiologists to detect more subtle initial presence, or more subtle interval changes in pattern and extent of ILD over time.30 When integrated with machine learning-based tools for detection, categorisation, and quantification, CT findings from ILD will be more accurate, and will allow for better and faster segmentation/quantification.
Better image quality with CT usually comes at the cost of increased radiation exposure, and the associated risks to the patient.31 This trade-off does not apply to PCCT, where a better image quality is obtained at a lower radiation exposure.32 This is because the spectral capabilities of PCCT allow it to identify low-energy scattered photons (noise). Removing noise from the system results in improved signal-to-noise performance of PCCT.27 Studies with use of PCCT demonstrate improved characterisation of ILD with radiation doses 28–32% lower compared to standard CT.33 The utility of low-dose HRCT techniques has been established with traditional CT systems.34 The additional denoising capabilities of PCCT may facilitate better-quality low-dose HRCT protocols, hopefully leading to increased utilisation of HRCT for surveillance of SARD-ILD.
CT Imaging Reconstruction
There has been a shift in CT image recon techniques utilising machine learning to improve the speed and quality of CT recons. These ‘deep learning image reconstructions’ (DLIR) provide a way to denoise CT images. Like PCCT, this results in improved image quality, and enables the use of lower radiation doses for HRCT. For example, Zhao et al.35 compared standard-dose, standard-recon HRCT with low dose HRCT (38% of dose of standard-dose exam) using DLIR, in a population of 70 prospectively enrolled patients with ILD. Despite the significant reduction in dose, the use of DLIR resulted in significantly better recognition of ground glass, architectural distortion, bronchiolectasis, and honeycombing.
The aggregate effects of the incorporation of both PCCT and DLIR into HRCT imaging techniques will have a multitude of benefits. Firstly, it will improve CT sensitivity, resulting in detection of more subtle disease (higher sensitivity) and more confident determination of ILD stability versus progression (hence, impacting management). Secondly, it improves accuracy of characterisation of CT findings related to ILD (more accurate imaging-based ILD pattern recognition and prognostication). Thirdly, lowering the cumulative radiation dose means less hesitation in use of HRCT for screening, diagnosis, and follow-up, especially for younger females, who make up a large portion of the SARD-ILD patient population.
CT: Artificial Intelligence
In recent years, there have been significant developments in the use of artificial intelligence (AI) for CT-based characterisation and quantification of ILD. Machine learning holds the promise of a more reproducible and quantifiable approach to what has traditionally been a very qualitative endeavour, the interpretation of HRCT. In this portion of the review, representative work in the areas of disease detection (screening), pattern recognition (diagnosis), and quantification (management) will be discussed. Although it is expedient to group the applications of AI this way, machine learning algorithms can be trained to ‘do it all’ at one point in time.
In the area of early detection, the merging of AI into CT technology dates back to 2011, when a computer program capable of detecting and quantifying ILD on HRCT was developed and validated in a patient cohort with familial pulmonary fibrosis or RA (with or without ILD).36 This program used segmentation, texture analysis, training, classification, and grading to score ILD, and was able to consistently identify mild ILD. In a recent study, a fully convolutional network was used for semantic segmentation of several ILD patterns.37 After training the algorithm, it was used to analyse HRCT data from two separate databases. Despite some limitations, the study proved the feasibility of creating a fully automated software for detecting the presence of pulmonary fibrosis.
ILD pattern recognition on HRCT is of prognostic value. For example, in patients with SARD-ILD, the presence of a usual interstitial pneumonia (UIP) pattern on HRCT is associated with decreased survival, compared to patients without typical UIP features.38 Recently, deep learning methodologies were innovatively tested to predict histopathologic diagnosis. A custom DL model was evaluated to explore the non-invasive diagnosis of UIP, and compared to histopathologic diagnosis. The researchers trained the DL model with 894 CT cases, where each case was assigned a label based on histopathologic diagnosis (UIP or non-UIP). The deep learning model was subsequently validated in another 198 cases. The model’s reproducibility was compared against intra-rater and inter-rater radiologist reproducibility. Deep learning performance was superior to visual analysis in predicting histopathologic diagnosis (area under the receiver operating characteristic curve: 0.87 versus 0.80, respectively; P<0.05) with a significantly greater reproducibility compared to radiologist inter-rater and intra-rater reproducibility39.
HRCT plays an important role in the ongoing care of patients with SARD-ILD, as serial quantification of HRCT findings assists in determining ILD behaviour as stable versus progressive. A post-processing tool called Computer-Aided Lung Informatics for Pathology Evaluation and Ratings (CALIPER) uses HRCT information, such as texture analysis, to identify and quantify CT features predictive of mortality and forced vital capacity decline.40,41 CALIPER increases radiologist confidence as to whether HRCT findings of SARD-ILD are stable or progressing on follow-up CT (Figure 2), which impacts management decisions. When a radiologist with access to CALIPER encounters a case where quantitative analysis may be useful, the axial thin supine reconstruction is sent to the CALIPER server. Within minutes, results are available with a map of the lung volume segmentation (upper versus mid versus lower, and central versus peripheral), a colour-coded reconstruction of the pulmonary findings, and a glyph summarising the distribution and relative abundance of findings, such as emphysema, normal lung, ground glass, reticulation, and honeycombing (Figure 3). Such representation of CALIPER-based parameters is shown to be better than traditional functional parameters for assessing outcomes and mortality in patients with SARD-ILD.42
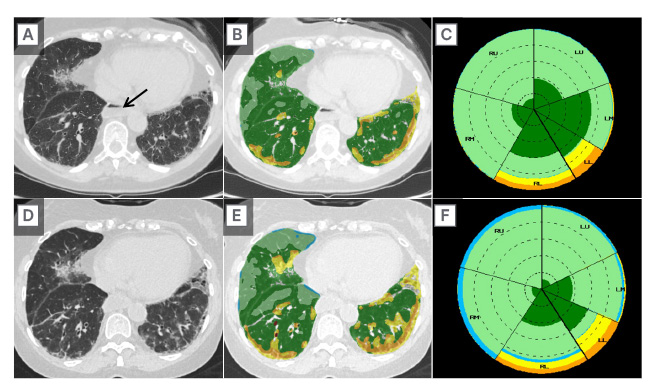
Figure 2: Application of artificial intelligence to high-resolution CT in assessing disease progression.
Baseline axial CT image (A); axial image with color-coded CALIPER overlay (B); and summary CALIPER Glyph (C) from a young female with systemic sclerosis and interstitial lung disease, compared to a 6-month follow-up HRCT (D, E, F).
Subjective comparison of the baseline (A) and follow-up (D) CT images suggests some possible ILD progression; however, the fact that the ILD has progressed is much easier to appreciate when comparing the colour-coded CALIPER images (B versus E). Note the patulous oesophagus (A, arrow), a classic CT finding for patients with SSc-ILD.
HRCT: high-resolution CT; ILD: interstitial lung disease; SSc-ILD: systemic sclerosis-related ILD.
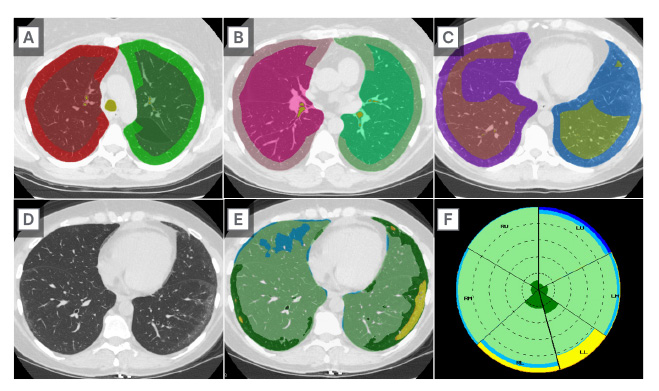
Figure 3: Characterisation of lung parenchymal changes using artificial intelligence incorporated high-resolution CT.
Colour-coded representative axial CT images from CALIPER demonstrating central versus peripheral segmentation in the upper (A), mid (B), and lower (C) lung zones. Axial CT images without (D) and with (E) colour-coded CALIPER overlay demonstrating subtle peripheral areas of ground-glass (yellow) in a patient with SARD-ILD. The darker green peripheral areas (E) often go on to develop into ground glass, suggesting future disease progression. A summary CALIPER Glyph (F) demonstrates the relative distribution of HRCT findings.
SARD-ILD: systemic autoimmune rheumatic disease-related interstitial lung disease.
Positron Emission Tomography-CT: New Tracers
The use of positron emission tomography with CT (PET/CT) to assess ILD activity has, thus far, been based on the use of 2-deoxy-2-[fluorine-18] fluoro-D-glucose PET with CT (FDG PET/CT). This tracer, while useful for a variety clinical applications, is limited to assessing the degree of inflammation rather than fibrotic activity/pulmonary remodelling in the setting of ILD.43 Persistent activation and local accumulation of myofibroblasts play a key role in the development of fibrotic diseases of the lung.44,45 Fibroblast activation protein α (FAP) is induced in activated fibroblasts, and is negligible in resting fibroblasts.
An advancement in molecular imaging is the development of radiolabelled quinoline-based PET tracers that bind to FAP, such as 68Ga-FAP-inhibitor (68Ga-FAPI), which demonstrate tracer uptake in various tumour entities, and in fibrotic diseases.46 A study in 21 patients with SSc-ILD reported the use of 68Ga-FAPI-04 PET/CT, and showed that FAP imaging directly visualised activated fibroblasts in vivo.47 Increased 68Ga-FAPI-04 uptake at baseline was associated with progression of ILD, independently of extent of involvement on HRCT scans, and of the forced vital capacity on baseline PFTs. Moreover, changes in 68Ga-FAPI-04 uptake were concordant with the observed response to the fibroblast-targeting antifibrotic drug nintedanib.47 These new PET/CT tracers may play an important role in managing SARD-ILDs in the near future.
MRI
MRI for evaluation of SARD-ILD, although desirable due to a lack of ionising radiation and due to superior tissue characterisation, has not become a mainstream tool for the diagnosis and management of ILD. Relative to HRCT, major drawbacks of lung MRI include lower spatial resolution (related to low proton density in lungs), and long acquisition times (resulting in respiratory motion artefacts exacerbated by poor breath-holding in the ILD population). Advances in lung MRI sequences, such as ultrashort echo time sequences, which are faster, with less signal decay and higher resolution, have increased investigation of MRI as a potential tool for imaging ILD.
Recently, ultrashort echo time MRI has shown near-perfect inter-method agreement compared to HRCT for ILD changes, in terms of assessing extent, severity, and pattern (reticulation, traction bronchiectasis, and honeycombing) of disease.48,49 An area of limitation with HRCT is the ability to consistently differentiate inflammatory ground-glass opacities (GGO) from fibrotic GGO. In this regard, MRI tissue characterisation has been shown to differentiate active alveolitis from fibrosis based on the higher water content in active inflammation. T2 weighted imaging helps identify active alveolitis by high T2 signal and early enhancement in patients with nonspecific interstitial pneumonia and UIP. On the other hand, fibrosis has low T2, and a more delayed pattern of enhancement.46 This MRI-based differentiation could potentially be a useful predictor of treatment response of active alveolitis. MRI could also aid in deciding the need for, and choice of, immunosuppressive therapy, depending on the presence or absence of alveolitis in chronic infiltrative lung disease.50,51
In specific SARD-ILDs, like SSc-ILD, recent studies have shown good agreement between lung MRI and HRCT in the assessment of ILD in SSc, with 100% sensitivity of MRI relative to HRCT.52 Lung MRI demonstrated good diagnostic performance and correlation with forced vital capacity and DLCO; however, MRI underestimated disease extent relative to HRCT.53 Short tau inversion recovery MRI, on the other hand, was a good indicator for disease progression independent of HRCT. In this same study, both T1 and sort tau inversion recovery values were significantly different between normal and abnormal lung parenchyma.54
The limitation of conventional MRI in evaluating the lung parenchyma is due to its low proton density, resulting in low contrast images of the lungs on magnetic resonance images. The magnetic resonance signal may be further degraded by air-tissue interfaces, respiratory motion, and blood flow in the vasculature.55 To overcome this limitation, functional MRI using hyperpolarised gases has been an advancement. For example, O2-enhanced MRI may aid early evaluation of ILD, and serve as a marker for disease progression.56 O2-enhanced MRI was found to be comparable to CT in SARD-ILD in assessing pulmonary function loss and disease severity.57 In another study, hyperpolarised helium 3 (3He) diffusion-weighted (DW) MRI was used to demonstrate reproducibility with chest CT findings of sub-pleural and basilar fibrotic changes in patients with ILD.58 Further, 3HeDW-MRI being a functional study, the elevated DW MRI metrics correlated with the diffusing capacity of the lungs for carbon monoxide, consistent with deteriorating alveolar gas transfer. MRI, with its detection of active inflammation, ability to predict response to treatment, and newer functional assessment tools, has a lot of potential for the evaluation of SARD-ILD.
Lung Ultrasound
Lung ultrasound (LUS) has been studied in the setting of ILD for more than a decade, and has the advantage of being radiation free, non-invasive, portable, and low cost.59 Convex and linear probes have both been used in LUS, with frequencies ranging from 3.5–10.0 MHz, depending on the transducer used. Limited scanning of the posterobasal chest, between the scapula and diaphragm, has been proposed as a sensitive and quick screening tool for ILD evaluation60.
B-lines and pleural abnormalities are the two main diagnostic criteria used in LUS for ILD.60 B-lines are reverberation artefacts, which are caused by the reflection of ultrasound waves by interlobar septal thickening from fibrosis. They appear as hyperechoic lines that extend from pleural surface to edge of field, and move synchronously with lung sliding. As a screening tool, B lines have a high sensitivity and negative predictive value.61 Total B-line cut-offs have been reported in SSc-ILD and RA-ILD with high sensitivity.62,63 Total B-lines have also significantly differentiated ILD versus no ILD, and anterior B-lines have differentiated extensive ILD from limited ILD.64 LUS as a follow-up tool has been shown to detect new onset ILD in known SSc and worsening ILD.65 In a patient with amyopathic dermatomyositis, a combination of LUS B-lines and KL-6 were applied successfully in the ongoing follow-up of a patient with known ILD on immunosuppressive therapy.66
Pleural abnormalities can be detected on LUS in the setting of ILD, and are easily identifiable and reproducible. In SSc-ILD, pleural abnormalities on LUS include pleural thickening (≥3 mm) and granularity, defects in pleural surface, or pleural irregularity. A preliminary prospective investigation using the pleural abnormalities for diagnosis of ILD had high sensitivity, specificity, and excellent inter-reader agreement.67 The sensitivity of B-lines increases when paired with pleural abnormalities for diagnosis.
A major limitation of LUS is that it cannot distinguish between the various types of pulmonary fibrotic findings, such as reticulation, GGO, or honeycombing; nor can it identify the pattern of fibrosis as nonspecific interstitial pneumonia or UIP.60 Also, using B-lines in the setting of ILD has its own limitations. B-lines can be a nonspecific sign of an interstitial process, and are seen with interstitial oedema. B-lines can also be seen in areas of decreased aeration, posing a problem for the evaluation of the lung bases.60 B-lines are difficult to quantify, and can be dependent on machine settings, which affect reproducibility. There is currently no consensus in LUS for definition of lesions, standard acquisition parameters, areas to be scanned, quantification, scoring systems, or transducers used. Overall, LUS can be a sensitive and accessible radiation-free screening tool for SARD-ILD, when in the right hands, but the modality needs further validation and standardisation with larger prospective studies.
Figure 1 depicts the potential future role of the imaging innovations reviewed in this manuscript in screening and monitoring of SARD-ILDs.
CONCLUSION
HRCT has been a pivotal imaging development in the past two decades, and has become the mainstay in the diagnosis of ILD in patients with SARDs. There have been significant innovations in imaging in the past decade, as described in this article, with compelling evidence to support the integration of these innovations into mainstream clinical practice. While larger scale validation of these modalities is required, the future holds promise in terms of costs without compromising quality.