Abstract
Vitamin D (VD) is a key factor in calcium-phosphorus metabolism. In addition, it has increasing popularity due to its pleiotropic effects: renal protection, antineoplastic properties, and diabetes mellitus and hypertension control. The VD axis is severely impaired in chronic kidney disease. The changes are present even in the earliest stages and progress as kidney function worsens. Significant changes in VD occur after successful kidney transplantation, as different factors interplay, leading to widespread VD insufficiency in kidney transplant recipients. The aim of our review is to demonstrate the changes in VD metabolism after kidney transplantation and to reveal their full impact on graft and patient survival in the post-transplant setting. Furthermore, current strategies for VD supplementation and their efficacy will be discussed.
THE VITAMIN D AXIS: IN HEALTH AND IN CHRONIC KIDNEY DISEASE
Vitamin D Metabolism in Healthy Subjects
Vitamin D (VD) and its metabolites are hormones and hormone precursors, synthesised predominantly (~90%) endogenously in the skin. The ultraviolet rays from the sun transform 7-dehydrocortisol (provitamin D) to previtamin D, which isomerises to cholecalciferol (vitamin D3) under the influence of body temperature. Oral intake also contributes to VD levels (≤10%), in the form of ergocalciferol (vitamin D2) and vitamin D3.1 Both substances have equivalent biological effectiveness. VD is activated by two-step hydroxylation, which occurs in the liver and kidneys. First, hydroxylation takes place in the liver, by the enzyme 25-hydroxylase (CYP27A1), and 25-hydroxyvitamin D (25VD) is synthesised. The final activation step occurs in renal tubules, where 25VD is hydroxylated to 1,25 dihydroxyvitamin D (1,25VD) by 1-α hydroxylase (CYP27B1). 1,25VD is the major active VD metabolite, as its binding capacity to the VD receptor (VDR) is almost 1,000-times higher than the other VD molecules.2 CYP27B1 is influenced by several factors. Serum calcium, phosphate, thyroid hormones, metabolic acidosis, fibroblast growth factor 23 (FGF-23), and 1,25VD suppress its activity, whereas prolactin, calcitonin, and somatotropin increase it.
25VD is the metabolite used to evaluate VD status due to its longer half-life (2–3 weeks). Apart from enzyme activity, sun exposure is a major factor influencing VD status, with the seasonal peak from July–September and the seasonal nadir from February–April.
VD generates its physiological effects via binding to the VDR. Thus, VD-reacting elements are activated, leading to the activation of genes, influencing calcium–phosphorus metabolism. As a result, intestinal calcium and phosphorus absorption is increased, bone remodelling occurs, parathyroid hormone (PTH) secretion is suppressed, and renal calcium and phosphate absorption is increased. These are the classical effects of VD.
VDR is detected in practically all human tissues. In addition, CYP27B1 activity was detected in other organs: intestinal epithelial cells, prostate gland, pancreas, and the central nervous system. These factors explain the possible properties of VD beyond calcium-phosphorus metabolism: renal protection, immunomodulation, and diabetes mellitus control. These non-classical effects are known as VD pleiotropy.3 Figure 1 summarises VD metabolism and its classical and pleiotropic effects.
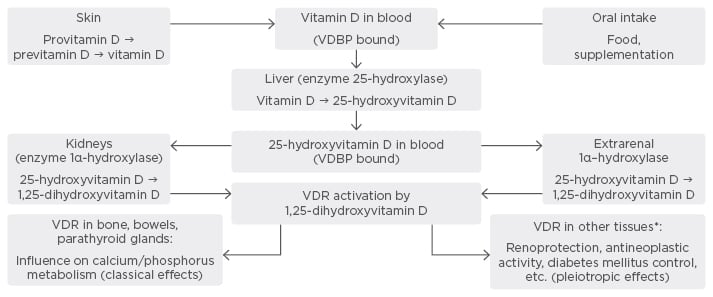
Figure 1: Metabolism and physiological effects of Vitamin D.
*Glomerular cells, immune cells, pancreatic islets cells, etc.
VDBP: vitamin D binding protein; VDR: vitamin D receptor.
Vitamin D Metabolism in Chronic Kidney Disease
Abnormalities in VD metabolism are detected in the early stages of chronic kidney disease (CKD).4 All components of the VD axis are affected: cholecalciferol, 25VD, 1,25VD, and the VDR. Table 1 summarises the VD abnormalities in kidney disease.
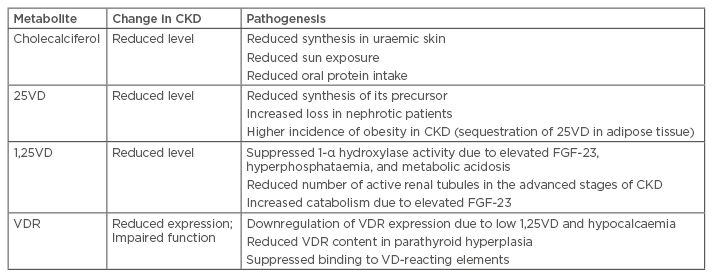
Table 1: Vitamin D metabolism in chronic kidney disease: findings and pathogenesis.
25VD: 25-hydroxyvitamin D; 1,25VD: 1,25-dihydroxyvitamin D; CKD: chronic kidney disease; FGF-23:
fibroblast growth factor 23; VD: vitamin D; VDR: vitamin D receptor.
CKD-related abnormalities in VD are part of a wider CKD-related syndrome: CKD-related mineral bone disorder (CKD-MBD), which includes biochemical changes (abnormalities in serum calcium, phosphorus, PTH, alkaline phosphatase, FGF-23, and VD), bone pathology, and extraskeletal calcium deposits. CKD-MBD is associated with increased risk for bone fractures, higher incidence of cardiovascular events, and increased mortality in CKD patients. CKD-MBD changes become more expressed with the progression of kidney disease.5
Vitamin D Metabolism After Successful Kidney Transplantation
Significant changes in mineral metabolism occur after successful renal transplantation. Successful kidney transplantation (KT) leads to rapid reduction of FGF-23 level within the first 3 months after the procedure.6 PTH levels rapidly decrease within the first 3 months, and remain stable and often elevated after the first post-transplant year.5 Hypophosphataemia and hypercalcaemia are common in the early post-transplant period and tend to normalise after the third month of KT.
It takes ≤18 months for VD status to improve after renal transplantation. However, despite the presence of a functioning graft, VD status in kidney transplant recipients (KTRs) is usually suboptimal. VD sufficiency was <~18% in the spring-summer period and dropped further to 2.59% in the winter period in our transplant centre, indicating the persistent influence of sun exposure on VD status even after renal transplantation.7,8 Similar rates were established in other transplant centres.9 The reasons for poorer VD status after KT are multifactorial, but can be classified as CKD-related or transplantation-specific. Moderate or advanced CKD (estimated glomerular filtration rate [eGFR] <60 mL/min/1.73 m2) is common after renal transplantation, reaching ≤70%.10,11 CKD-related issues are outlined in Table 1.
Transplant-specific factors include limiting sun exposure in order to reduce the risk for skin malignancies, immunosuppressive treatment (steroids, calcineurin inhibitors [CNIs]), new onset diabetes after transplantation (NODAT), and a higher incidence of obesity after KT. Table 2 summarises the transplant-specific factors that have an impact on VD status.
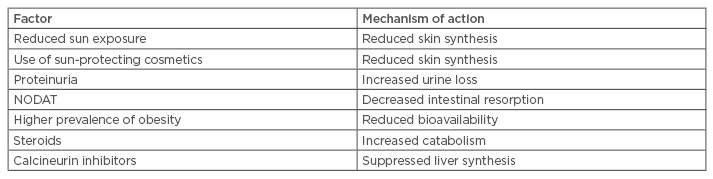
Table 2: Transplantation-associated factors influencing vitamin D status after kidney transplantation.
NODAT: new onset diabetes mellitus after transplantation.
Importance of Vitamin D in the Post-Transplant Period
The two major clinical issues concerning VD after KT are mineral-bone disease and VD pleiotropy.
Post-transplant mineral bone disease
Post-transplant mineral bone disease (PTr-MBD) consists of the same three components as CKD-related MBD (biochemical abnormalities, bone involvement, soft tissue calcification) and is similarly associated with increased fracture risk and death. Poor VD status is one of the factors for developing PTr-MBD, together with immunosuppressive therapy, persistent hyperparathyroidism, malnutrition, persistent CKD, duration of CKD Stage 5, hypogonadism, metastatic cancer disease, smoking, duration of dialysis and transplantation, obesity, and diabetes mellitus.
Biochemical abnormalities in post-transplant mineral bone disease
Biochemical findings are highly dependent on pre-transplant CKD-MBD, immunosuppressive treatment, post-transplant graft function, concomitant diseases, and medications. Apart from suboptimal levels of VD, all other factors in calciumphosphorus metabolism can also be persistently dysregulated. PTH levels remain significantly increased ≤43% after the first year after KT.7 Similar results were observed in our institution, with hyperparathyroidism reaching 33.69% in Bulgarian KTRs with a KT duration >12 months. Post-transplant PTH and FGF-23 abnormalities are associated with higher incidence of hypophosphataemia and hypercalcaemia, though they tend to resolve after the first year.12 An immunosuppressive regimen also indirectly influences mineral metabolism. Steroids suppress calcium intestinal absorption and increase VD catabolism. In addition, our findings suggest that CNIs are associated with lower 25VD levels, probably due to impaired liver synthesis.8
Mineral–bone indicators should be monitored closely immediately after KT. The frequency of testing for calcium, phosphorus, PTH, alkaline phosphatase, and 25VD should be determined according to graft function, duration of transplantation, magnitude of abnormalities, and when supplementation was initiated. An optional follow-up for CKD-MBD indicators was suggested by Kidney Disease: Improving Global Outcomes (KDIGO) for CKD patients and KTRs.5 In our centre, a similar approach was adopted. However, 25VD levels are monitored at least twice annually, taking into consideration its seasonal variations. Thus, significant deterioration of VD status in the winter/fall was detected, allowing adequate supplementation to be initiated.
Post-transplant bone disease
A rapid reduction in bone density is widely reported, with faster bone loss during the first months after successful KT, though reduced bone density loss was reported years after the operation. A major complication is increased fracture risk, associated with increased morbidity and mortality. The factors contributing to post-transplant bone disease have already been listed. Pre-transplant bone health is crucial for post-transplant outcomes. A key factor in deteriorating bone health prior to kidney transplantation are excessive VD doses, which are associated with increased incidence of adynamic bone disease before KT. Therefore, adequate treatment of CKD-related MBD should be performed in the pre and post-transplant period.
Vascular calcification
Assessing the development of soft-tissue calcification is difficult due to the high prevalence of vascular calcification in advanced CKD. Only one study demonstrated possible slowing of the calcification process after KT.13 Established risk factors for vascular calcifications were statin use, low 25VD levels, male sex, older age, and higher phosphate levels.14 In addition, immunosuppressive treatment has multifaceted influence on vascular health. Mycophenolate mofetil proved to have protective effects against calcification, especially compared to steroids and CNIs.15 The mTOR-inhibitor rapamycin was found to inhibit smooth muscle cell proliferation, thus reducing the risk for vascular calcification. Everolimus impaired the vasoactive and antithrombotic function of the endothelium.16 Therefore, due to the contradictory reports, relatively scarce scientific data dealing with this problem, and difficulties in quantification of vascular calcification, this topic remains open for further discussion.
Vitamin D pleiothrophy after kidney transplantation
Short-term graft survival has improved significantly over the years, peaking at 98% survival for the first 12 months after KT. However, long-term graft survival is lagging behind: 10-year survival ranges between 60% and 70%. The reason for these poorer results are NODAT, interstitial fibrosis/tubular atrophy, and patient death due to cardiovascular disease (CVD) or neoplasia.
Due to the wider distribution of the VDR, VD is associated with several pleiotropic effects: renal protection, improving blood pressure, improved glycaemic control, antineoplastic effect, suppression of the renin-angiotensin-aldosterone system (RAAS), and immunomodulatory properties. Thus, we can expect that VD may solve the above-mentioned problems and improve post-transplant outcomes. These findings, however, were detected in the general population or CKD patients. The data for KTRs are relatively scarce and are limited to single-centre reports and retrospective studies.
Vitamin D and renal protection after transplantation
Lower 25VD levels were associated with poorer graft function and faster GFR decline.17,18 Poorer VD status was associated with worse kidney function in the long run too.19 A possible explanation for poorer graft survival in lower 25VD is increased rejection rate.20 In addition, proteinuria is associated with faster decline of the graft function. Similarly to studies in the non-transplant population, we demonstrated that poorer VD status was associated with higher proteinuria after KT.21 The possible antiproteinuric mechanisms of VD are RAAS suppression, nuclear factor κΒ inactivation, Wnt/β-catenin pathway suppression, and upregulation of slit-diaphragm proteins.
In animal models, treatment with the VDR activator paricalcitol reduced cyclosporine toxicity.22 Data from interventional studies in humans are still scarce and controversial. Cholecalciferol supplementation was not effective in modifying proteinuria and interstitial fibrosis/tubular atrophy after KT. Similarly, the single-centre randomised controlled trial (RCT) Vita-D failed to demonstrate renoprotective and immunomodulatory effects in KTRs supplemented with cholecalciferol.23,24 Contrary to cholecalciferol supplementation, paricalcitol significantly reduced proteinuria in KTRs.25
Vitamin D and new onset diabetes after transplantation
The association between poorer VD status and diabetes mellitus has been reported in the general population.26 In KTRs the evidence for VD–NODAT association is still uncertain. NODAT is associated with higher morbidity and mortality after transplantation, and is linked to the use of steroids and CNIs. However, a report demonstrated a link between its prevalence and VDR genetic polymorphism.27 Observational data did not demonstrate better glycaemic control in diabetic DM in our transplant centre. Unfortunately, no interventional studies were reported that assessed the VD-NODAT relationship.28
Vitamin D and cardiovascular disease after transplantation
The risk for CVD is increased after transplantation compared to the general population, due to the persistent CKD-associated vascular and cardiac abnormalities, especially vascular calcifications. Poor VD status was associated with arteriosclerosis and endothelial dysfunction in end-stage renal disease patients. In addition, it was related to increased CVD incidence in poor VD status. An alternative explanation for CVD incidence in low VD levels is that VDR activation in cardiomyocytes suppresses their proliferation. However, the studies after solid organ transplantation are insufficient and inconclusive. As already mentioned, low 25VD is an established risk factor for vascular calcifications after KT.13 Other reports did not demonstrate an association between CVD and VD insufficiency in KTRs.29 Furthermore, higher doses of VD may be associated with increased risk for vascular calcifications.30
Vitamin D and rejection
VDR is expressed in all immune cells, including those of the innate immune dendritic cells and macrophages. Therefore, significant influence of VD on the immune system can be expected. Calcitriol was found to suppress T and B-lymphocyte proliferation; it inhibits dendritic cells and macrophages, suppresses interleukin and immunoglobulin G production, and downregulates major histocompatibility complex class II expression. Apart from experimental studies, there are a small number of studies in KTRs. A recent study indicated that better VD status is associated with lower incidence of acute rejection.18 Horwedel et al.31 detected a trend for lower acute cellular rejection incidence after high dose VD supplementation was applied, without reaching statistical significance.31 An observational study also supported the protective effect of VD against acute rejection.20 However, initial data from the Vita-D study do not reveal any positive effects from cholecalciferol supplementation.24 In addition, a recent single-centre study also failed to establish a link between rejection and VD status in Canadian transplant recipients.32 Evidently, there are conflicting results concerning this issue and larger RCTs are needed to evaluate the VD–rejection relationship.
Vitamin D and infection
Infection represents a major cause of patient death and graft loss after successful kidney transplantation. VD was established to upregulate the synthesis of the lysosome protein cathelicidin and the anti-microbial protein β–defensin. Several RCTs in the general population indicated that better VD status was associated with lower incidence of viral, bacterial, and fungal infections.33,34
The reports in patients with solid organ transplantation are controversial. Poorer VD status was associated with higher incidence of opportunistic pulmonary infections in KTRs and lung transplant patients.35,36 Contrary to these findings, cholecalciferol supplementation did not improve the infection rate after renal transplantation in the Vita-D study.24 VD status had no influence on bacterial urinary tract infection rate in Bulgarian KTRs followed up in our centre.37 However, Kwon et al.38 determined VD status as an independent risk factor for urinary tract infections after transplantation. Currently, RCTs evaluating the association between infection risk and VD status after KT are lacking.
Vitamin D and malignancy
Neoplasia is one of the most common causes for patient and graft loss in KTRs. VD was found to suppress cellular proliferation and angiogenesis, and stimulates cell differentiation and apoptosis. In addition, VD reduces the metastatic potential of neoplasia via improving intercellular adherence. Several human studies indicate that better VD status is associated with lower incidence of colorectal cancer, breast cancer, and non-Hodgkin lymphoma.39-41 However, in certain carcinomas both higher and lower serum 25VD levels are linked to increased incidence, e.g. prostate and pancreatic cancer.42,43
There have been several conflicting reports concerning the antineoplastic property of VD after KT. Poorer pre-transplant VD status was related to higher post-transplant cancer incidence; supplementation with active VD also reduced the malignancy risk in KTRs.29,44 Contrary to these findings, another study did not find associations between neoplasia and VD status.45 In order to fully understand the association between neoplasia and VD status, interventional studies are of crucial importance, as other pro-neoplastic factors exist after KT.
Vitamin D and mortality
All-cause mortality is increased in KTRs, due to persistent CKD, persistent cardio-vascular disease, post-transplant mineral bone disease, and increased neoplastic incidence and infection rate. Sub-optimal VD was associated with higher all-cause mortality in non-CKD and CKD patients.46,47 Similar findings were established for KTRs: VD deficient recipients (VD <25 nmol/L) had increased mortality.48 Again, prospective RCTs after KT are needed.
Vitamin D Treatment in Kidney Transplant Recipients
These are the main indications for VD treatment: native VD (cholecalciferol/ergocalciferol), active VD metabolite, or VDR agonists. Treatment should be tailored to the initial VD status after KT, as well as the post-transplant calcium–phosphorus metabolism, due to the increased incidence of hypercalcaemia and hypophosphataemia after successful KT. The therapy should follow the trends in the mineral-bone metabolism; therefore, the values for calcium, phosphorus, alkaline phosphatase, and PTH should be evaluated regularly. The frequency of laboratory testing should be based on graft function, degree of biochemical abnormalities, and supplementation initiated. Generally, the recommendations suggested by KDIGO are similar to those for non-transplant CKD patients and can be changed if stricter control is warranted.5 Currently, data are present for the treatment of post-transplant MBD-related biochemical indicators during the first 12 months. There are no sufficient data for the influence of supplementation on fracture risk and VD pleiotropy in KTRs.
Cholecalciferol/ergocalciferol supplementation
Native VD effectively reduced PTH levels after KT.49 The influence of VD supplementation on bone density is controversial. Sahin et al.50 reported reduced bone loss in patients supplemented with cholecalciferol and calcium, whereas these findings were not confirmed by Wissing et al.51 Therefore VD status should be assessed in all KTRs and supplementation is recommended with doses similar to those for the general population.5 However, cholecalciferol/ergocalciferol treatment increases the risk for hypercalcaemia. In addition, we are waiting for the results from two large prospective trials with native VD supplementation in KTRs: VITALE and CANDLE-KIT, which will assess the effect of supplementation on graft function, NODAT incidence, rates of acute rejection, cancer incidence, and mortality rates.52,53
Treatment with calcitriol/VDR activators
Calcitriol was found to effectively suppress PTH levels and improve bone density after KT.54 Paricalcitol significantly reduced PTH and proteinuria and improved bone density in KTRs.25,55 Furthermore, paricalcitol treatment was not associated with increased risk for hypercalcaemia. More RCTs are needed to elucidate the effect of active VD or VDR activators on bone density and their pleiotropic effects.
CONCLUSION
Significant changes occur in the VD axis in CKD and after kidney transplantation. The importance of these changes spans beyond calcium-phosphorus metabolism and may have a direct impact on graft and patient survival. Larger prospective and interventional RCTs are needed to fully assess the influence of VD on post-transplant outcomes.