Abstract
Diabetic kidney disease (DKD) is defined as a clinical syndrome consisting of persistent macroalbuminuria, progressive decline in glomerular filtration rate (GFR), hypertension, increased cardiovascular disease events, and the associated mortality of these conditions. The disease evolves from the microvascular complications of poorly controlled Type 1 diabetes mellitus (T1DM) and Type 2 diabetes mellitus (T2DM). The pathogenic pathways comprise renal haemodynamic changes, ischaemia and inflammation, and overactive renin–angiotensin–aldosterone system (RAAS), through which several events cascade down from hyperglycaemia to renal fibrosis. Conventional and novel renoprotective strategies target modifiable DKD risk factors and specific stages of the pathogenic pathways, respectively. Although these strategies may slow DKD progression to end-stage kidney disease (ESKD), novel drugs are still undergoing trials for validation in human participants. This narrative review appraises these renoprotective strategies and highlights the current clinical staging and pathogenesis of the disease.
INTRODUCTION
Type 1 diabetes mellitus (T1DM) is predominant in childhood and adolescence whereas Type 2 diabetes mellitus (T2DM) is more common in adulthood. Nevertheless, the prevalence of T2DM is rising in children and adolescents as well.1 The major predisposing factor in both developed and developing countries is the increase in cases of paediatric obesity and metabolic syndrome.2 Both types of diabetes are associated with long-term microvascular complications following poor glycaemic control, as defined by glycosylated haemoglobin or haemoglobin A1c (HbA1c) level of >7.5%.3 These complications include diabetic nephropathy, diabetic retinopathy, and diabetic neuropathy.
Diabetic nephropathy progresses to chronic kidney disease (CKD) over 10–20 years.4 Microalbuminuria (urine albumin excretion rate of 30–299 mg/day or albumin/creatinine ratio of 30-299 mg/g creatinine) is the first biomarker to appear. It may regress to normoalbuminuria or progress to macroalbuminuria (urine albumin excretion rate of >300 mg/day or albumin/creatinine ratio of >300 mg/g creatinine). Macroalbuminuria signals the development of overt diabetic kidney disease (DKD), which is characterised by a progressive reduction in the estimated glomerular filtration rate (eGFR) to <60 mL/minute/1.73 m2, resulting in end-stage kidney disease (ESKD). Thus, DKD is defined as a clinical syndrome comprising persistent macroalbuminuria (noted at least twice within a 3–6-month interval), progressive decline in eGFR, hypertension, increased cardiovascular disease events, and the associated mortality of these conditions.5,6
DKD represents a significant cause of morbidity and mortality in patients with diabetes, and is the leading cause of ESKD on a global scale.7,8 Although advanced DKD takes decades to develop, its structural lesions may manifest within 1.5–5 years in child and adult patients.⁹ The structural lesions in diabetic children and adults with normoalbuminuric DKD are similar. Patients with T1DM may present with albuminuria without changes in eGFR. In contrast, adults with T2DM and micro- or macroalbuminuria progressively manifest typical DKD structural lesions as the eGFR declines.9 The classical stages of DKD in T1DM are absent in adults with T2DM, given their frequent comorbidity with hypertension, albuminuria, and kidney failure at diagnosis. Conventional renoprotective strategies target modifiable DKD risk factors in diabetic children and adolescents, identified as diabetes duration,10 hypertension,11 dyslipidaemia,12 obesity,13 and smoking.14 These factors are linked to microalbuminuria; however, none of these renoprotective strategies can stop or reverse DKD progression.15 More importantly, some of them at best can retard the rate of decline in renal function. Given the transition of 25% of normoalbuminuric diabetic patients to CKD,16,17 decline in renal function remains the most critical target of renoprotection.18 The diverse pathophysiologic mechanisms of renal injury in diabetes are still unfolding. Thus, novel drugs for DKD are emerging and are still undergoing clinical trials. This narrative review appraises the renoprotective strategies for DKD in childhood and adolescence. Additionally, it highlights the current clinical staging and pathophysiologic mechanisms of the disease.
CLINICAL STAGING AND PATHOPHYSIOLOGIC MECHANISMS OF DIABETIC KIDNEY DISEASE
Different hypotheses on the pathophysiologic mechanisms of DKD have been advanced.19-21 It is now believed that renal fibrosis constitutes the final common pathway arising from hyperglycaemia-induced renal haemodynamic changes, oxidative stress, inflammation, hypoxia, and overactive renin–angiotensin–aldosterone system (RAAS).6 In fact, DKD is defined by the changes in renal structure and function in diabetes. The structural changes include mesangial expansion, basement membrane thickening of the glomeruli and tubules, and glomerulosclerosis. The functional changes affect urine albumin excretion, eGFR, and blood pressure. Thus, the clinical stages consist of glomerular hyperfiltration, normoalbuminuria, microalbuminuria, and ESKD.22 Stage 1 is characterised by glomerular hyperfiltration, increased eGFR, increased urine albumin excretion rate, and normal blood pressure. In Stage 2, thickened glomerular basement membrane and mesangial expansion, normal eGFR, normoalbuminuria, and normal blood pressure occur. Stage 3 presents with microalbuminuria, declining eGFR, and hypertension, whereas Stage 4 presents with macroalbuminuria, further reduction in eGFR to <60 mL/minute/1.73 m2, and hypertension. Stage 5 is marked by ESKD, decreasing albumin excretion rate, and hypertension. Nevertheless, a recent proposal described the clinical stages as follows: Stage 1 (from diabetes onset to 5 years) is characterised by borderline eGFR, no albuminuria or hypertension, a 20% increase in renal size, and elevated renal plasma flow.23 Stage 2 (from 2 years after onset) is identified by normal eGFR and no clinical symptoms.23 In Stage 3 (5–10 years after onset), glomerular damage and microalbuminuria occur with or without hypertension, whereas in Stage 4, irreversible proteinuria, sustained hypertension, and eGFR <60 mL/minute/1.73 m2 are seen.23 Stage 5 refers to ESKD with eGFR <15 mL/minute/1.73m2.23 This staging is not different from the previous staging, underscoring the current unanimity in its categorisation. DKD is a complex disease linking haemodynamic and metabolic pathways with oxidative stress and systemic inflammation.22 The pathways (renal haemodynamic changes, ischaemia and inflammation, and overactive RAAS) through which several events cascade down from hyperglycaemia to renal fibrosis are shown in a schematic diagram (Figure 1). The novel DKD drugs target specific stages of these pathways. Firstly, the renal haemodynamic pathway comprises the following sequence of events: hyperglycaemia induces the release of vasoactive mediators (insulin-like growth factor-1 [IGF-1], glucagon, nitric oxide [NO], prostaglandin, and vascular endothelial growth factor [VEGF]) which cause the dilatation of the glomerular afferent arterioles.⁶ Additionally, the high filtered glucose load from hyperglycaemia triggers the upregulation of sodium-glucose transporter-2 (SGLT2), leading to increased tubular reabsorption of glucose and sodium chloride. Consequently, reduced availability of sodium chloride at the macula densa occurs; afferent arteriolar dilatation from tubuloglomerular feedback and efferent arteriolar constriction from elevated local angiotensin II levels both contribute to glomerular hypertension.23 Insulin resistance, hyperinsulinaemia, and hyperglycaemia independently lead to endothelial dysfunction by producing reactive oxygen species (ROS), activation of protein kinase C, and proinflammatory signalling from advanced glycated end-products. Specifically, compensatory hyperinsulinaemia increases endothelin-1 secretion, which causes vasoconstriction and vascular dysfunction.24 In summary, renal endothelin-1 A receptor activation leads to vasoconstriction, podocytopathy, oxidative stress, inflammation, and fibrosis.25
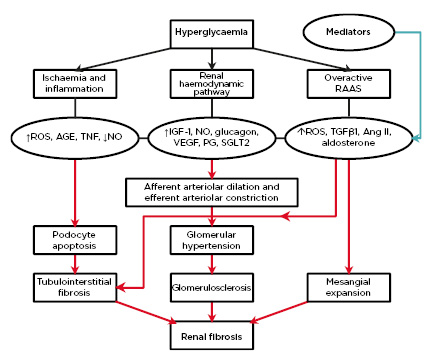
Figure 1: A schematic diagram of the major pathophysiologic mechanisms in diabetic kidney disease.
AGE: advanced glycated end-products; Ang II: angiotensin II; IGF-1: insulin-like growth factor-1; NO: nitric oxide; PG: prostaglandin; RAAS: renin-angiotensin-aldosterone system; ROS: reactive oxygen species; SGLT2: sodium-glucose transporter-2; TGFβ1: transforming growth factor β1; VEGF: vascular endothelial growth factor.
Secondly, the events in the ‘ischaemia and inflammation’ pathway are triggered by the direct and indirect effects of hyperglycaemia.⁶ Hyperglycaemia directly triggers the production of ROS, which contributes to podocyte apoptosis. Also, hyperglycaemia-induced cellular stress leads to proinflammatory responses and the activation of NF-kB by ROS and TNF. NF-kB is linked to proteinuria and infiltration of inflammatory cells into the renal interstitium.26 Again, the glomerulosclerosis in DKD is associated with renal medullary hypoxia. Advanced DKD leads to reduced intrarenal production of NO.27 Failure to supply the compensatory increase in oxygen demand by residual functional nephrons results in the generation of nephrotoxic free radicals. Hyperglycaemia also impairs the stability of the reactively increased hypoxia-inducible factor meant to ameliorate the hypoxic state, and thus promotes renal tissue fibrosis.
Thirdly, hyperglycaemia and advanced glycated end-products trigger the renal expression of renin and angiotensinogen through ROS, as well as the kidney-specific G protein-coupled metabolic receptor GPR91.28,29 Overactivity of RAAS worsens DKD deterioration via mediators such as transforming growth factor-β1 (TGFβ1), angiotensin II, and aldosterone, which are all associated with renal tissue fibrosis.30,31 In fact, inhibition of RAAS retards the progression of DKD;32 a finding which supports the role of overactive RAAS in disease pathogenesis.
BIOMARKERS IN DIABETIC KIDNEY DISEASE
To achieve effective renoprotection, DKD should be identified early enough with biomarkers. The traditional biomarkers of glomerular injury such as albumin/creatinine ratio and eGFR can predict DKD. However, both biomarkers may lack specificity and sensitivity for the following reasons. Firstly, not all patients with microalbuminuric T1DM and T2DM will progress to the late stages of DKD because many of them may have normoalbuminuric DKD. Secondly, glomerular biomarkers (e.g., TNF-α, transferrin, Type IV collagen, and lipocalin-type prostaglandin D synthase) and tubular biomarkers (e.g., neutrophil gelatinase-associated lipocalin, nephrin, N-acetyl-β-D-glucosaminidase, and liver-type fatty-acid binding protein) appear before microalbuminuria,33 which suggests that albumin/creatinine ratio can only predict the late stages of DKD. Moreover, tubulointerstitial lesions occur earlier than glomerular injury in diabetic nephropathy, showing that tubular biomarkers are more sensitive.
In fact, there are disadvantages in using microalbuminuria and eGFR as measures of renal function decline. For instance, there is a daily variation in urine albumin/creatinine ratio and eGFR values may be affected by the patient’s haemodynamics, diet, and hydration status, and become more sensitive only in advanced DKD.34 Predicting DKD at an early stage when renoprotective strategies would make a difference remains a diagnostic challenge. Thus, the limitations of albumin/creatinine ratio have resulted in a paradigm shift to novel molecular biomarkers which have higher sensitivity and specificity for earlier prediction of DKD. Using the ‘multi-omics’ methods (transcriptomics, proteomics, lipidomics, and metabolomics) to profile patients’ bio-specimens (renal biopsy, plasma, and urine samples) has led to the recent identification of candidate biomarkers.34
RENOPROTECTION IN DIABETIC KIDNEY DISEASE
Renoprotection in DKD involves risk-specific interventions against disease progression. Despite its drawbacks, albuminuria remains the most important prognostic biomarker for the progression of CKD.15 There are modifiable and nonmodifiable risk factors for microalbuminuria and its progression to macroalbuminuria in children and adolescents with T1DM. The risk factors for microalbuminuria include female sex, hypertension, duration of diabetes, high normal urine albumin excretion, elevated low-density lipoprotein-cholesterol (LDL-C) and triglycerides, high BMI, and smoking.10-14 On the other hand, the risk factors for macroalbuminuria comprise male sex, elevated HbA1c, high serum uric acid, and smoking.35-38 Adolescents with T2DM present a different picture regarding DKD risk factors. These factors appear more prevalent and less amenable to control in T2DM compared to T1DM. For instance, hypertension, dyslipidaemia, and obesity are more common in patients with T2DM than in their T1DM counterparts.39-41 Interventional measures in DKD consist of correcting hyperglycaemia, hypertension, and dyslipidaemia, as well as lifestyle modification.42 Primary renoprevention circumvents the progression of normoalbuminuria to microalbuminuria. Secondary renoprevention involves the prevention of macroalbuminuria from microalbuminuria. Thus, conventional renoprotective strategies are mainly directed against the modifiable risk factors for microalbuminuria and its progression to macroalbuminuria. Also, novel drugs with different mechanisms of action are emerging as effective treatment strategies (Table 1). Additionally, multiple interventions like strict glycaemic control, blood pressure control, correction of dyslipidaemia, and lifestyle change (such as smoking cessation) led to an improvement in the prognosis of cardiovascular disease events and retarded the progression of macroalbuminuria in patients with T2DM and DKD.43
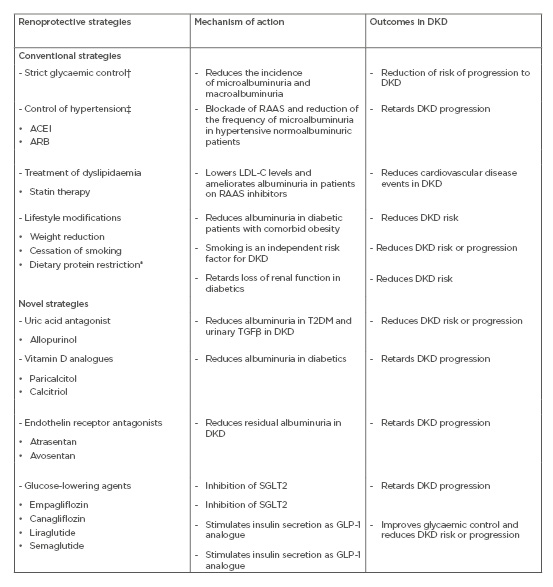
Table 1: Some conventional and novel renoprotective strategies in diabetic kidney disease.
ACEI: angiotensin-converting enzyme inhibitor; ARB: angiotensin-receptor blocker; DKD: diabetic kidney disease; GLP-1: glucagon-like peptide-1; HbA1c: haemoglobin A1c; KDIGO: Kidney Diseases Improving Global Outcomes; LDL-C: low-density lipoprotein-cholesterol; NKF: National Kidney Foundation; RAAS: renin-angiotensin-aldosterone system; SGLT2: sodium-glucose transporter 2; TGFβ: transforming growth factor β.
CONVENTIONAL RENOPROTECTIVE STRATEGIES
Strict Glycaemic Control
The efficacy of strict glycaemic control as a renoprotective strategy depends on the severity of DKD given its non-beneficial effect in advanced stages of the disease. In studies of patients with T1DM, strict glycaemic control (maintaining HbA1c level at 7%) substantially reduced the incidence of microalbuminuria and macroalbuminuria.44,45 A meta-analysis also indicated that strict glycaemic control in patients with T1DM for 8–60 months decreases DKD progression risk.46 In patients with T2DM, the same strategy resulted in a lower risk of microvascular complications compared to dietary treatment47 and delayed the onset of microalbuminuria and macroalbuminuria, despite failing to reduce the incidence of ESKD.48 Other strategies are thus required in synergy with strict glycaemic control to reduce the burden of DKD. Concurrent medical control of hyperglycaemia, hypertension, dyslipidaemia, and microalbuminuria significantly delayed progression to overt DKD, retinopathy, and ESKD in microalbuminuric patients with T2DM.42,49 Thus, glycaemic control should be individualised according to the patient’s comorbidities, such as underlying CKD and cardiovascular disease status. Although the Kidney Disease Outcomes Quality Initiative (KDOQI) recommends that strict glycaemic control should keep the HbA1c level to <7% in patients with T1DM and T2DM, a HbA1c level of >7% is suggested as the target for those with multiple comorbidities and risks of hypoglycaemia episodes.50 Regarding response to glycaemic control, children and adolescents with T2DM appear to differ from adults with T2DM. For instance, the Treatment Options for Type 2 Diabetes in Adolescents and Youth (TODAY) study suggested that T2DM and its complications may be much more severe and resistant to glycaemic control in children and adolescents compared to their adult counterparts.51 This multicentre trial specifically showed failure of glycaemic control in 46% of paediatric trial participants despite adequate compliance with metformin alone, metformin and rosiglitazone, or metformin and intensive lifestyle management.
Control of Hypertension
Blood pressure control is effective in retarding DKD progression, reducing cardiovascular disease events, and preventing early mortality in patients with T1DM and T2DM.22 It reduces microalbuminuria and delays the onset of DKD.52 RAAS blockade reduces the frequency of microalbuminuria in hypertensive normoalbuminuric patients. This reduction does not occur in normotensive patients because intrarenal RAAS is enhanced in hypertensive patients but not in normotensive patients.6 There is a direct relationship between hypertension and the degree of albuminuria in T2DM.53 Thus, RAAS blockade confers benefits extending beyond mere blood pressure control in hypertensive patients with diabetes. Angiotensin-converting enzyme inhibitors (ACEI) or angiotensin-receptor blockers (ARB) are recommended as first-line antihypertensive drugs, especially in patients with albuminuria. A study group reported that ACEI reduced the risk of DKD in patients with T1DM with persistent microalbuminuria.54 A similar therapy in normotensive and normoalbuminuric patients with T1DM failed to slow the progression to DKD.55 Besides ACEI/ARB, calcium-channel blockers can serve as an alternative antihypertensive therapy, either alone or in combination with RAAS blockers. Again, findings from the TODAY study indicate that blood pressure control was more difficult in children and adolescents with T2DM compared to their adult cohorts. Elevated blood pressure in the former was less amenable to treatment, requiring multiple drugs in >33% of trial participants who were initially on monotherapy.56 However, clinical trials have revealed some therapeutic challenges in adults. The Veterans Affair Nephron Diabetes study reported no significant difference between ACEI/ARB combination therapy and ACEI or ARB monotherapy, as risks of hyperkalaemia and acute kidney injury could increase with the combination therapy.57 Additionally, the Ongoing Telmisartan Alone and in Combination With Ramipril Global Endpoint Trial (ONTARGET) showed that combination therapy with telmisartan and ramipril reduced macroalbuminuria to normoalbuminuria but resulted in renal function decline, increased requirements for renal replacement therapy, and increased mortality risk.58
Treatment of Dyslipidaemia
Statin therapy may ameliorate albuminuria and reduce cardiovascular disease risk in patients with DKD. For instance, in a trial of atorvastatin versus rosuvastatin, the former showed more renoprotective effects than the latter in reducing albuminuria in patients with DKD receiving RAAS blockers.59 Additionally, the KDOQI guideline suggests that patients with DKD should receive statin-based medications to lower the levels of LDL-C and reduce the risks of cardiovascular disease events.50 Nevertheless, a comparison between the response of adult and paediatric patients with T2DM to hypolipidemic therapy suggests a difference in treatment outcomes. In the TODAY study, sustained elevation in inflammatory markers was noted over time and dyslipidaemia increased from 4.5 to 10.7% in paediatric patients over 3 years of follow-up, despite hypolipidemic therapy.60
Lifestyle Modifications
Weight reduction, smoking cessation, and dietary restriction of sodium and protein constitute the bedrock of lifestyle modifications. Weight reduction in patients with diabetes with comorbid obesity led to a marked reduction in albuminuria.61 The Kidney Disease Improving Global Outcomes (KDIGO) guideline recommends the avoidance or cessation of smoking for patients who have DKD or are at risk of DKD because it is deemed an independent disease risk factor.62 Dietary modification is also useful for retarding the progression of DKD. Although the optimal sodium intake in DKD remains unresolved, its restriction reduces blood pressure and albuminuria.63 Finally, protein restriction retards the progressive loss of renal function in T1DM and T2DM.64 The KDIGO and the National Kidney Foundation (NKF) recommend a target dietary protein intake of 0.8 g/kg/day for people with diabetes and those with reduced eGFR.65 Furthermore, adequate exercise can help to reduce DKD risk and cardiovascular disease events in diabetic adolescent and adult patients in whom improvements in blood pressure, lipid profile, insulin sensitivity, and cardiovascular disease outcomes were reported.66,67
NOVEL RENOPROTECTIVE STRATEGIES
Uric Acid Antagonist
Allopurinol, a uric acid antagonist, is effective in reducing albuminuria in T2DM.68 The mediatory role of uric acid in the pathogenesis of DKD is the basis for using this agent as a renoprotective intervention. Hyperuricaemia leads to glomerular endothelial dysfunction, overactive RAAS, and induction of inflammatory pathways, which all contribute to the renal lesion in DKD.69 Thus, it is not surprising that allopurinol also improves endothelial dysfunction and reduces urinary TGF-β1 in DKD.70
Vitamin D Analogues
The pleiotropic effects of vitamin D receptor activation underscore the potential usefulness of vitamin D analogues in DKD. Given the presence of vitamin D receptors on podocytes, podocyte modulation with these metabolites is thought to affect proteinuria. In some clinical trials, paricalcitol reduced albuminuria in patients with diabetes, and retarded the progression of DKD.71,71 Vitamin D metabolites may also be renoprotective by inhibiting RAAS and preventing glomerulosclerosis.
Endothelin-Receptor Antagonists or Inhibitors
Endothelins modulate hypertension and CKD through the mechanisms of cell injury, vasoconstriction, endothelial dysfunction, and albuminuria. Two endothelin receptors are involved: endothelin-1 A and endothelin B receptors. The latter mediates proximal tubular sodium excretion. Endothelin receptor antagonists such as atrasentan and avosentan have undergone trials and were found to reduce albuminuria in DKD.25,73 Avosentan was, however, associated with cardiovascular adverse events, possibly because it blocked both endothelin A and B receptors, unlike atrasentan that selectively inhibited endothelin-1 A receptors and was associated with minimal fluid retention.
Glucose-Lowering Agents
Apart from glycaemic control, some novel glucose-lowering agents may be renoprotective as well. For instance, several recent clinical trials indicated that SGLT2 inhibitors (empagliflozin and canagliflozin) may be associated with slower progression of DKD in T2DM patients.74,75 Similarly, glucagon-like peptide-1 (GLP-1) analogues (liraglutide and semaglutide) resulted in the same renal outcomes in patients with diabetes.6 GLP-1 can trigger insulin secretion and mediate glycaemic control. Importantly, its level is reduced in patients with T2DM. Thus, its analogues are beneficial in improving disease morbidity especially in adult patients with T2DM.
Other glucose-lowering agents with their specific therapeutic targets include linagliptin and saxagliptin (dipeptidyl peptidase 4 inhibitors which preserve the glucagon-like peptide effect), and rosiglitazone (thiazolidinediones which activate peroxisome proliferators-activated receptor-γ to increase tissue insulin sensitivity).6 These drugs reduced albuminuria in macroalbuminuric patients with T2DM.76-78
FUTURE RESEARCH DIRECTIONS
Proteomics and metabolomics have identified potential biomarkers to monitor DKD and its progression and provided information on disease pathophysiologic mechanisms.79-82 More research on these ‘multi-omics’ methods will provide clinicians with the best approach to manage DKD in the future. Finally, bioinformatics have enabled the identification of noncoding RNA as potential biomarkers and therapeutic targets given their important role in DKD progression.83 Investigating the regulatory network of these noncoding RNA will open a new outlook for understanding the molecular mechanisms in DKD and how the transcriptomes can act as novel biomarkers and potential therapeutic targets for DKD.83
CONCLUSIONS
DKD evolves from diabetic nephropathy, a critical microvascular complication of T1DM and T2DM. Although the late stages of DKD rarely occur in the paediatric age group, structural changes suggestive of the disease may manifest as early as 1.5–5.0 years after the onset of diabetes in both child and adult patients. Thus, conventional and novel renoprotective strategies may help to retard the progression of DKD to ESKD in children and adolescents. Despite the evidence-based successes reported with most of these strategies, some of the novel therapeutic agents are still undergoing trials to validate their use in human participants.