Abstract
The increasing prevalence of arboviral diseases, driven by urbanization and travel, coupled with the absence of specific treatments or vaccinations, has led to a growing burden on global health. Despite the implementation of vector control programs, outbreaks continue to occur. While vaccines are considered the ultimate solution, progress in the development of vaccines for dengue virus, Zika virus, Chikungunya virus, and West Nile virus has been limited, due to factors such as limited access to target populations, lack of funding, and concerns about safety and efficacy across all age groups. Furthermore, the growing trend of vaccine hesitancy presents a significant barrier to future vaccine adoption. This review provides a subtle overview of current progress in the development of vaccine candidates for these prevalent arboviral diseases.
Key Points
1. Arboviruses have shown pandemic potential due to their ability to rapidly spread across different regions and cause significant morbidity and mortality. With increasing globalization and climate change, the risk of arboviral outbreaks and pandemics is a growing concern for global health security.
2. This manuscript focuses on the latest progress in the development of vaccines against arboviruses, shedding light on preclinical and clinical investigations aimed at evaluating the safety, immunogenicity, and efficacy of these vaccine candidates. Additionally, the manuscript delves into the challenges of vaccine production and potential impact on vaccine hesitancy for arboviral prevention.
3. The progress in vaccine technology has accelerated the development of novel arbovirus vaccines. Some vaccine candidates have shown promising results, but further research is necessary to guarantee their safety and efficacy. Collaborative efforts involving researchers, policymakers, and public health agencies play a crucial role in accelerating vaccine development, obtaining regulatory approval, and facilitating global vaccine distribution.
INTRODUCTION
The importance of vaccines in the fight against infectious diseases cannot be emphasized enough. Vaccine technological improvements in recent decades have led to the production of a variety of effective vaccines against infectious diseases.1 Unfortunately, vaccinations for many diseases, such as arboviral infections, are still unavailable.1 Arboviruses account for roughly 17% of the worldwide burden of infectious disease, with a mortality rate of 700,000 deaths per year.2 These infectious diseases, caused by viruses such as dengue virus (DENV), Zika virus (ZIKV), West Nile virus (WNV), and Chikungunya virus (CHIKV), have no specific treatment, and only a few vaccines are available to prevent them.1,3
Arboviruses are spread by arthropod vectors (mosquitoes and ticks), and have recently risen from obscurity to a public health emergency of international concern.4 Recent arbovirus epidemics have been closely connected to urbanization, international travel, and climate change.5 Because therapeutic alternatives have not been supportive or specific, the attention has mostly been on techniques that aid in the removal of arbovirus vectors, such as insecticide-based strategies, which reduce disease burden. To cope with arboviruses and lessen the overall burden of arboviral diseases, technologies concentrating on diagnostic tools, serological testing, antivirals, and vaccines are now being developed. This review intends to investigate promising vaccine options for selected arboviral diseases, while also addressing vaccine production obstacles.
ARBOVIRAL DISEASES AS A GLOBAL HEALTH PROBLEM
Arboviruses of global significance are endemic in certain areas, particularly in the tropics, and the majority of infections caused by these viruses demonstrate asymptomatic or non-pathognomonic symptoms, such as fever, malaise, and headache.6 Some of the most noteworthy arboviruses of worldwide significance in the tropical region include ZIKV, DENV, CHIKV, and WNV.7
Dengue Virus
DENV, a Flaviviridae family member, has four serotypes, and is responsible for the majority of arboviral infections each year.1 DENV infection has spread over the Americas, Africa, Europe, Southeast Asia, the Eastern Mediterranean, and the West Pacific. Annually, approximately 390 million cases are reported, with a quarter of these instances presenting with a mild, severe, or deadly disease. According to research, over 4 billion people in 129 countries are at risk of DENV infection.8,9
DENV infections can result in mild illness or an asymptomatic clinical course. A large proportion of people infected with DENV experience a vague, generally benign, and self-limited course, with up to half of them remaining asymptomatic.10 DENV is rarely detected in individuals without diagnostic testing. Individuals under the age of 15 years, or those who have DENV for the first time, are more likely to show mild symptoms. These individuals fully recover without requiring hospital care. After 2–7 days of a high fever of up to 40 °C (104 °F), and at least two other symptoms, adults and children over the age of 15 are frequently diagnosed with classic dengue fever.10
Severe headache, diffuse maculopapular erythematous rash, retro-orbital eye pain, myalgia, arthralgia, and mild hemorrhagic signs are common symptoms. A high fever caused by dengue can be especially dangerous because of the individual’s increased risk of seizures and other neurological impairments. Subconjunctival mucosa, buccal mucosa, soft and hard palate, and easy bruising of the lower limbs are common hemorrhagic symptoms.11 Epistaxis, gingival bleeding, gastrointestinal bleeding, and urogenital bleeding are possible complications of dengue fever. Laboratory tests frequently reveal leukopenia with lymphocyte predominance and thrombocytopenia to varying degrees. These individuals may also suffer from severe nausea and vomiting, which can lead to dehydration.11,12
Zika Virus
Before its initial epidemic in 2007 on Yap Island in the Federated States of Micronesia, ZIKV, another member of the Flaviviridae family, had only produced occasional cases in Africa and Asia.1 By 2013, the virus had spread to French Polynesia and other Pacific countries and territories, causing a large-scale pandemic in Brazil 2 years later.13,14 Over the years, at least 86 countries and territories in the Americas, Africa, Asia, Oceania, and Europe have reported over 3,500 cases of ZIKV infection.15,16
Infection with ZIKV has been associated with congenital malformations and immunological diseases.17 ZIKV is frequently self-limiting, with an estimated incubation time of only a few days after being bitten by an infected mosquito.18 The majority of the initial cases were documented in people between the ages of 20–40 years, but there were a few outliers in people as young as 4 months, and as old as 98 years.19
The primary symptom of this condition is maculopapular erythematous exanthema, which begins on the face or trunk and spreads to the limbs, palms, and soles. The rash caused by CHKV infection was very similar to this one. Low-grade fever, exudative conjunctivitis, myalgia, arthralgia, headaches, and other symptoms like those of DENV and CHKV infections may occur. Individuals who test positive for ZIKV have also been linked to a variety of neurological disorders, including Guillain–Barré syndrome and meningoencephalitis.20,21
Chikungunya Virus
CHIKV, an Alphavirus of the Togaviridae family, was first identified in Tanzania in 1952.22 CHIKV virus has spread to at least 60 nations and territories throughout the world since 2004. Although the majority of patients recover well, outbreaks of this disease can be spontaneous.23 Symptoms of the disease can last for months, become chronic, and even result in death.
One of the most recent outbreaks occurred in the Republic of Chad, where the World Health Organization (WHO) received reports of around 27,540 cases from July–September 2020.24 Incubation time for CHKV infection ranges from 1–12 days, with an average of 2–4 days.25
The term ‘chikungunya’ was first used to describe the illness, which is derived from the acute onset of bilateral migrating arthralgia, which commonly affects the tiny joints of the fingers, wrists, toes, and knees, and ranges in severity from mild irritation to complete incapacitation. Arthralgia and arthritis usually affect multiple joints in a symmetrical pattern.26 The clinical course is usually mild and self-limiting, lasting 7–10 days; however, the arthralgia that follows can last for years. In addition to arthralgia and arthritis, infection can cause fever, myalgia, headaches, and, on rare occasions, a maculopapular, pruritic rash.26
Furthermore, CHKV can cause arthritis and has a quicker onset than DENV. This aids in differentiating the disease from DENV and ZIKV, particularly when it affects hands and feet.27 DENV and ZIKV have slower onset times, and cause more severe arthralgia. Given that children typically have a more self-limiting infection, these more severe symptoms are more commonly seen in older adults.27 Death from CHKV infection is uncommon, but likely, and it most commonly affects older adults due to cardiopulmonary failure or neuroinvasive illness.
West Nile Virus
WNV is another Flaviviridae family member that infects birds, mosquitoes, horses, humans, and other mammals.28 Incidences have been documented on all seven continents. Over 30,000 instances of human infections were documented in the first 5 years of the pandemic in North America.29 Although most of those infected are asymptomatic, the virus infection can proceed to cause neurological problems, which can result in death.30
Most individuals who are infected develop a mild illness, and less than 1% develop neurological symptoms, such as encephalitis, meningitis, meningoencephalitis, acute flaccid paralysis, ataxia, extrapyramidal signs, polyradiculitis, seizures, and eye nephritis.31 WNV is the most common cause of pandemic neuroinvasive illness in humans, and accounts for 1.4% of all central nervous system infections.32
WNV has spread throughout North America as a seasonal endemic infection since its discovery in 1999 in New York, USA. Between 1999–2017, the Centers for Disease Control and Prevention (CDC) reported 48,183 cases of WNV infection, including 22,999 cases of neuroinvasive illness.33,34 The mortality rate of individuals with neuroinvasive illnesses ranges from 8–12%.35
VACCINE DEVELOPMENT FOR ARBOVIRUS DISEASES
Arboviruses are medically important viruses that cause widespread infection around the world, especially in areas where their vectors are endemic, such as the tropics, subtropics, and Gulf Coast region.36,37 Several arbovirus vaccines are now being researched and tested in clinical trials, but only a few are commercially available.38,39 For example, a DENV vaccine in Phase III trials has shown varied vaccination efficacy against different serotypes, age, and previous exposure, and has been recommended for humans in epidemic areas.38
Arboviral infections have reportedly spread widely due to the current trend of rapid urbanization and increased worldwide commerce travels. As a result, there is an increasing demand for safe and effective arboviral vaccines. However, choosing the right vaccine vector is critical for successful vaccine development.38,40 Because there are currently no particular antiviral treatments for arboviruses, as well as limitations in vector control, vaccine development remains the most efficient means of protection.39
Dengue Virus Vaccines
DENV vaccines are now being developed worldwide, with live virus-vectored vaccines, live attenuated vaccines, nucleic acid vaccines, recombinant protein vaccines, and pure formalin-inactivated vaccines among the current development platforms.41 Complex disease etiology, resulting in varying levels of disease severity, lack of established correlates of protection, continually changing virus landscape, and lack of dependable animal model are all challenges impacting development platforms.42
Primary infection usually confers sole immunity against the infecting serotype, but infection by other serotypes is still a possibility, and it can be fatal.43 As a result, DENV vaccine development is solely focused on providing simultaneous protection against all serotypes of the virus.43 Even though several different DENV vaccines are in various phases of clinical development, Dengvaxia (Sanofi Pasteur, Lyon, France), a chimeric virus with the backbone of the yellow fever vaccine, strain YF-17D, is one of the most advanced developments in vaccines against dengue, and the only licensed DENV vaccine.41,44,45 The vaccine efficacy studies varied by age, serostatus, and serotype.46,47 Phase I and II trials to evaluate safety, immunogenicity, and reactogenicity were conducted in several countries in healthy adults ranging in age from 18–45 years. More than 30,000 people participated in Phase III trials, including children aged 2–16 years.48 Although it was deemed safe and well tolerated in a three-dose regimen, with no vaccine adverse effects, the absence of dengue virus non-structural proteins in the vaccine mix, however, predisposes seronegative persons to a higher risk of severe dengue infection.46
In recent years, studies have shown an increased frequency of hospitalization and severe dengue in vaccinated seronegative persons. This is because the vaccination resembles the underlying infection, and reinfection manifests as severe dengue.46,49 Additional vaccine risk studies demonstrated that the vaccine follows a distinct path that results in immunity in individuals who are seropositive at the time of inoculation.42 As recommended by the WHO, vaccination should involve a pre-immunization screening technique, in which only patients who are dengue seropositive are chosen for vaccination.46
However, Dengvaxia was also reportedly approved by the U.S. Food and Drug Administration (FDA) for use in children aged 9–16 years who live in dengue endemic areas, and have a prior laboratory-confirmed infection.50 In the midst of Dengvaxia complications caused by the potential for severe disease in dengue naïve individuals, good vaccination risk management could aid in the prevention of antibody-dependent enhancement induced severe vascular leakage.51 Dengvaxia administration could also help prevent transmission during epidemics, lowering the likelihood of severe disease.51
TAK-003 (Takeda Pharmaceuticals, Osaka, Japan) is one of the few vaccines that has recently completed Phase III trials.47 A live attenuated DENV-2 strain and three chimeric viruses with membrane and envelope protein genes from DENV-1, DENV-3, and DENV-4 strains expressed on the backbone of the DENV-2 genome make up the vaccine.47 Immunogenicity was found to be lower in seronegative people who got one primary dosage compared with those who received multiple doses in Phase II trials.46
The TAK-003 vaccine proved less serostatus dependent than Dengvaxia after two doses in Phase III studies, with more promising vaccination efficacy. However, the vaccine’s potential utility and safety are still being debated due to complex nuances for DENV-3 and DENV-4 strains.46 Another DENV vaccine that has completed Phase III trials is the National Institute of Health’s (NIH; Bethesda, Maryland, USA) live attenuated tetravalent DENV vaccine TV003/TV005.52 The vaccine consists of DENV-1, DENV-3, and DENV-4 that have been genetically attenuated, as well as a chimeric fourth component made up of structural genes from DENV-2 inserted into DENV-4.47 The vaccine’s immunogenicity is identical to that seen in populations with natural immunity, and a single dosage was found to be 100% effective in a human challenge study.46 However, despite the vaccine’s more optimistic concept than Dengvaxia and TAK-003, data on the full analysis of Phase III trials are yet to be published (Table 1).
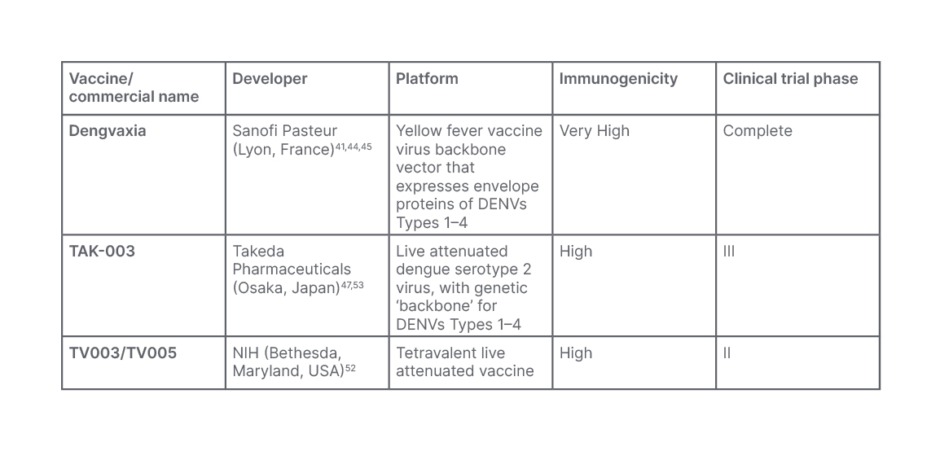
Table 1: Dengue vaccine candidates.
DENV: dengue virus; NIH: National Institute of Health.
Evidently, since a tetravalent immune response is required for immunity from all serotypes, individuals who are dengue naïve remain susceptible to infection due to antibody dependent enhancement; thus, the immunological interaction between four serotypes remains the most significant barrier to dengue vaccination.54
In the search for solutions to DENV vaccine challenges, two promising strategies have been proposed: sequential immunization and heterologous prime-boost strategy.46 According to reports, sequential immunization is significantly more immunogenic, and induces higher levels of neutralizing antibodies to all serotypes of DENV than tetravalent vaccine.55 Animal data from a study involving Singapore mice showed that sequential immunization demonstrates a diverse immunological profile, and could be offered as an alternative solution for the viral interference challenge of DENV vaccine.55 Nonetheless, a heterologous prime-boost strategy involving vaccine combination could provide another option.46
Dengvaxia significantly induces higher levels of protection against DENV serotypes 3 and 4 compared with serotypes 1 and 2, whereas TAK-003 significantly induces higher levels of protection against DENV serotypes 1 and 2.56 The varying serostatus dependence of both vaccines could also be factored. Since Dengvaxia’s dominant serotypes are the weakest for TAK-003 and vice versa, a prime-boost strategy involving administration of TAK-003, which is dominant against serotypes 1 and 2, followed by Dengvaxia, which is dominant against serotypes 3 and 4, is theoretically possible to counter viral interference, and offer broader immune responses against all serotypes.54
Zika Virus Vaccines
A significant number of ZIKV vaccine candidates are being developed, and are currently being tested in clinical trials. This includes a messenger RNA vaccine, as well as a DNA vaccine that encodes the ZIKV precursor membrane (prM) and E genes, and is currently being tested in Phase IIb trials in the USA, Brazil, Puerto Rico, Mexico, Panama, Peru, and Columbia. There have been several efforts to develop ZIKV vaccines that are both safe and effective.57
Purified inactivated viruses, live attenuated viruses, virus-like particles, recombinant viral vectors, DNA, messenger RNA, and viral proteins have all been the focus of these efforts.57,58 Some vaccines based on DNA and messenger RNA technologies that express prM/E protein, or purified inactivated viruses are currently undergoing clinical trials as ZIKV immunogens.59 DNA vaccines may be quickly developed using genetic engineering, and several targets on the viral structure that can be used as epitopes for eliciting immune responses.59 However, because of its history with clinical trials and effectiveness with yellow fever virus vaccines, the pure inactivated vaccine platform is regarded as the most effective.60 A lot of the vaccines were assessed using animal models utilizing non-human primates or immunosuppressed mice.58 A purified inactivated vaccine adjuvanted with alum was shown to be immunogenic after two doses by the intramuscular route in Phase I clinical trial, and a chimeric measles virus vaccine expresses the prM and E genes of ZIKV.61
Stephenson et al.62 demonstrated that ZIKV purified inactivated vaccines are safe and well tolerated by humans, but further clarity on the dosage required for adequate immunogenicity is still needed. When tested on mice, the GMZ-002 purified inactivated virus vaccine developed by Oh et al.63 also showed significant promise. When two doses of the vaccine were given, there were significant levels of serum neutralizing antibodies, a strong innate response, and undetectable viremia, albeit no human phase trials to replicate these results have been conducted yet.63 The CRM-EDIII-PM vaccine, developed by He et al.,57 is another intriguing contender. The vaccine could generate a substantial humoral and cellular immune response without causing major organ damage, although no human phase trials have been conducted so far.57
Currently, nine ZIKV vaccines are identifiably in clinical trial phases. There are three for purified inactivated viruses: ZPIV, developed by the Walter Reed Army Institute of Research (WRAIR; Silver Spring, Maryland, USA); PIZV, developed by Takeda Pharmaceuticals; and VLA-1601, developed by Valneva (Saint-Herblain, France). There are also three DNA vaccines: GLS-5700, developed by GeneOne/Inovio (Seoul, South Korea, and Plymouth Meeting, Pennsylvania, USA, respectively); and ZKADNA085-00-VP (1 and 2), developed by the Vaccine Research Center (VRC; Bethesda, Maryland, USA). There are two messenger RNA vaccines: mRNA-1325, developed by Moderna (Cambridge, Massachusetts, USA), and measles vectored MV-Zika, developed by Themis (Mumbai, India).58 Except for ZKADNA085-00-VP-2, which has progressed to Phase II, all of these vaccines are in Phase I. According to reports, all DNA vaccines have been approved for human testing.58
There are still a few obstacles to overcome before a safe and effective ZIKV vaccine can be licensed. Although most of these vaccination studies are still in the early phases, ZIKV transmission has decreased to the point where a Phase III efficacy study will be challenging to conduct (Table 2). A typical vaccine efficacy study would necessitate a large number of participants to account for confirmed cases in both symptomatic and asymptomatic individuals, as well as control groups.71 Although, the efficacy endpoints for a ZIKV vaccine in clinical trials are not well defined; one would wonder if a vaccine would have to prevent infection to prevent microcephaly, which is a very high bar for any vaccine.72 Nonetheless, the clear purpose of vaccines should be the prevention of congenital disease caused by the virus. As with previous outbreaks, efforts to develop vaccines diminish as the epidemic recedes.71
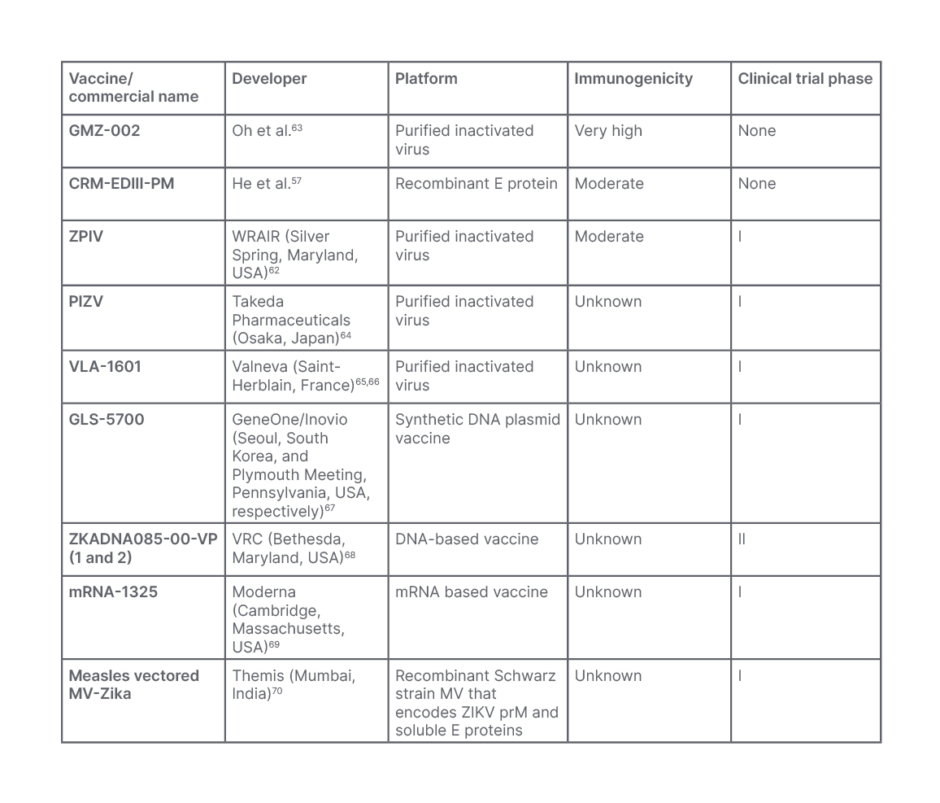
Table 2: Zika virus vaccine candidates.
mRNA: messenger RNA; MV: measles-vectored; prM: precursor membrane; VRC: Vaccine Research Center; WRAIR: Walter Reed Army Institute of Research; ZIKV: Zika virus.
Another impediment is the possibility of vaccine-mediated antibody dependent enhancement. Immunity to the ZIKV via vaccines may increase the risk of severe dengue due to cross-reactive antibodies from both viral diseases. It is critical that vaccine candidates elicit cross-reactive antibodies that are strong enough to provide protection, while avoiding vaccine-mediated antibody-dependent enhancement effects.71 Other challenges for ZIKV vaccine development include the lack of well-characterized animal models relevant to human disease, an incomplete understanding of immune responses required to prevent fetal infection, and the risk that immune responses from vaccines will induce Guillain–Barré and ZIKV-related pregnancy complications.73
Chikungunya Virus Vaccines
Diverse CHIKV vaccine candidates are being developed using various technologies, but the search for the best balance of safety and immunogenicity is still ongoing.74 There are currently no licensed CHIKV vaccinations; however, it is anticipated that one vaccine will protect against all CHIKV strains.75 When the formalin-inactivated vaccine was stopped due to production costs and safety concerns in the twentieth century, the USA military produced a live attenuated vaccine. Despite the latter’s encouraging results, further research was halted due to the USA military’s low priority.75
Research on CHIKV vaccines has been slow in recent years; however, a few replication-defective and attenuated vaccine candidates have shown promise in Phase I/II trials, and others have demonstrated safe and immunogenic in mouse and non-human primate models. Rossi et al.76 evaluated the immunogenicity and efficacy of a measles virus-vectored CHIKV vaccination in macaques, finding that all macaques tolerated the vaccine well with promising seroconversion rates. The study’s findings back up earlier Phase I and II clinical trial results.76 Reisinger et al.77 and Ramsauer et al.78 conducted a similar study with human participants, with the results confirming the vaccine’s outstanding safety and tolerability, as well as good immunogenicity.
Virus-like particles vaccines are also being developed, and are in clinical trials. These vaccines elicit neutralising antibodies against envelope proteins from various CHIKV strains and immunized non-human primates produced high titer neutralizing antibodies that protected immunodeficient mice from lethal infection.79 Nucleic acid-based vaccines are also showing promise in terms of production, safety, and the ability to produce humoral and cell-mediated immunity. Despite this, the majority of vaccines created have demonstrated minimal immunogenicity.79 Currently, eight CHIKV vaccines are in late pre-clinical or clinical development: TSI-GSD-218 (181/clone25), developed by the United States Armed Forces; VRC-CHKVLP059-00-VP (PXVX0317 CHIKV-VLP), developed by the NIH; MV-CHIK, developed by the Pasteur Institute (Paris, France); VLA1553, developed by Valneva; VAL-181388, developed by Moderna; pMCE321, developed by the University of Pennsylvania (Philadelphia, USA); CHIKV/IRES, developed by Takeda Pharmaceuticals; and EILV/CHIKV, developed by the University of Texas Medical Branch (Galveston, USA; Table 3).79
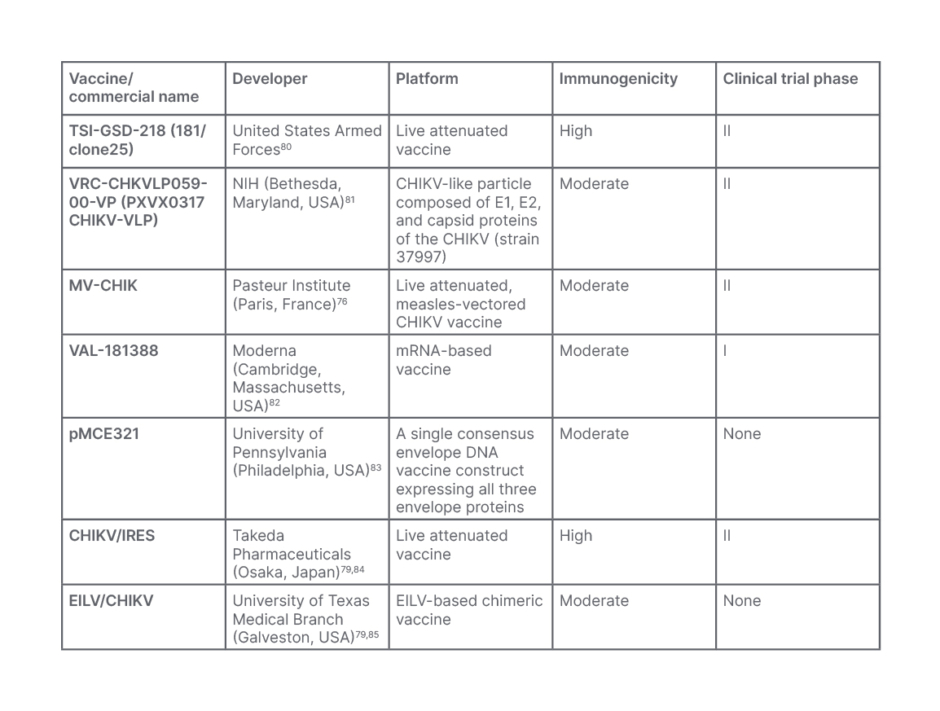
Table 3: Chikungunya virus vaccine candidates.
CHIKV; Chikungunya virus; EILV: Eilat virus; mRNA: messenger RNA; NIH: National Institute of Health.
Given that the CHIKV appears to be linked to big epidemics every 10–30 years, a return on investment is not guaranteed, and so the pharmaceutical sector has little incentive to produce the vaccines.61 These difficulties are exacerbated by policymakers’ difficulty in selecting how to roll out CHIKV vaccination during and between epidemics, as well as the difficulty of finding a good location for clinical efficacy studies during interepidemic periods. The unpredictable nature of viral epidemics further complicates the collection of human efficacy data.79 Randomized controlled trials, the gold standard, are not feasible during interepidemic periods due to the low expected cases. Similarly, the development of herd immunity, as well as the focal and episodic nature of CHIKV epidemics, have made Phase III clinical trials focusing on vaccine efficacy and safety logistically difficult.86
Antibody-dependent enhancement is another concern for CHIKV vaccine candidates. The risk of antibody-dependent enhancement potentially exacerbating viral symptoms cannot be completely dismissed, especially given the possibility of the emergence of new quasi-species harboring mutations or co-infection/re-infection with genetically related viruses, such as DENV, Ross River virus, or ZIKV, which can lead to more severe disease complications.87 Due to low levels of maternal-acquired anti-CHIKV antibody, escape mutants could infect infants due to the infection of pregnant females. Immune senescence in elderly patients may also have an impact on vaccine efficacy, and should be taken into account during vaccine development.83
West Nile Virus Vaccines
Several WNV vaccines have been proven to have high efficacy in animals, yet only a few of these vaccine candidates have been studied in humans. Unfortunately, the majority of them have only got to Phase I trial, and no human WNV vaccines have been authorized as of yet.88,89 Vaccine candidates for WNV include nucleic acids, virus-like particles, subunit elements, and recombinant viruses, as well as platforms based on pure inactivated and live attenuated viruses.89
A vaccine candidate using plasmid DNA expressing WNV prM/E was studied in the Phase I trial. The initial candidate, VRC-302 (Vical, San Diego, California, USA), and the later VRC-303 (Vical) utilize Cytomegalovirus promoter and a Cytomegalovirus promoter with an additional R region, respectively, to enhance gene expression.90 Although these vaccines, notably the VRC-303, produced a significant immune response and have high efficacy, the progress of these vaccines’ clinical trials has not been disclosed.90
The recombinant E protein vaccine (WNV-80E [Hawaii Biotech, Honolulu, USA]), which was also tested in Phase I clinical trials, showed promising results, but there are no reports on long-term antibody titers or vaccine efficacy in those over 45. HydroVax-001 (Oregon National Primate Research Center [ONPRC], Hillsboro, USA), the most promising WNV candidate evaluated in Phase I clinical trials, was recently revealed to have significant immunogenicity: however, the vaccine’s antigenic integrity would likely need to be improved.90
The formalin-inactivated WNV vaccine, and the most thoroughly investigated ChimeriVax-WN02 (Sanofi Pasteur) vaccine were the only candidates studied in Phase II clinical trials. The former lacks plausible immunogenicity evidence, but the latter has been claimed to be safe and efficacious, with better seroconversion rates. Even though none of the vaccine candidates revealed any safety problems or side effects that could jeopardise future clinical trials, the ChimeriVax-WN02 is thought to be the closest to licensure of all the vaccine candidates evaluated (Table 4).90,91
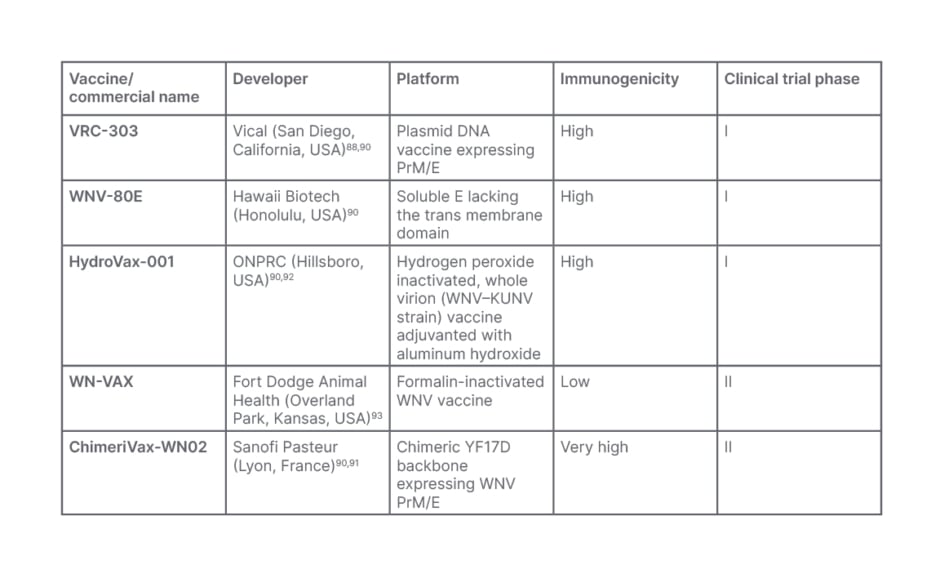
Table 4: West Nile vaccine candidates.
KUNV: Kunjin virus; ONPRC: Oregon National Primate Research Center; prM: precursor membrane; WNV: West Nile virus; YF17D: yellow fever virus 17D.
Several issues, such as the relatively short WNV season (May/June–September/October), and the sporadic occurrence of outbreaks, particularly in areas where there is co-circulation with other cross-reactive flaviviruses, make clinical trials difficult.89,90 Other factors influencing vaccine development are safety concerns, significant immunogenicity after a single dose, and the ability to distinguish between infected and vaccinated individuals.89
The cost per vaccine dosage is also expensive due to low case numbers, making vaccine production unnecessary. Although targeted immunization boosts cost-effectiveness, the population would still be less than that of other vaccines.91 More targeted vaccination efforts would be encouraged as the endemic area, case numbers, and evidence of genetic variants associated with severe types of viral infection grow.91 Nonetheless, the emergence of new antigenic variants as a result of mutations could be detrimental to vaccine development efforts.89 WNV genetic variants associated with severity would necessitate targeted vaccination approaches, which would undoubtedly impact production costs.91
CHALLENGES OF VACCINE PRODUCTION
Despite the identification of several potential candidates, vaccine development against arboviruses has had mixed success. One of the major limitations is a lack of detailed understanding of the vaccine’s inherent characteristics, which has a significant impact on the development of adaptive immunity against arboviral infection.94 For an optimal arbovirus vaccine, certain criteria must be met, including a single dose, long-lasting protection, a wide range of administration options, safety, and storage logistics.95 The significance of antigen selection for the production of subunit vaccines cannot be overstated; vaccine candidates’ important functions during the viral replication process substantially influence their immunogenicity and overall protective immunity.59
Three major elements drive the development of arboviral disease vaccines: necessity, availability, and cost. The number of documented acute or chronic cases can be used to determine the level of need, either worldwide or locally. Availability is determined by a suitable arrangement for commercial manufacture on a large enough scale to produce a sufficient and inexpensive supply that covers production costs and generates a profit. The question of whether activities are more likely to be pursued by industry or subsumed within the global public health structure is inextricably linked to the cost of development and the return on investment.96 Since arboviruses primarily affect developing countries, developing and manufacturing vaccines for emerging diseases is generally much more difficult from a financial and business standpoint than developing and manufacturing vaccines for diseases that are currently prevalent in developed countries.58
As we lack effective preventive and therapeutic options for these arboviruses, it is expected that we develop a reactionary approach that would accelerate the production of effective vaccines.97 The disinclination towards vaccine development until there is an outbreak could have a devastating impact, and production of an epidemic rushed vaccine could even have a disastrous impact due to a lack of rigorous evaluation and quality control.97
POTENTIAL IMPACT ON VACCINE HESITANCY FOR ARBOVIRAL PREVENTION
Nearly one-third of the 500 arboviruses discovered are linked to human diseases.98 Over the years, arbovirus vector control using insecticide-based methods had been the most common way of preventing arboviral diseases. Although, the alarming rates of insecticide resistance among mosquito populations stimulated new approaches through increasing understanding of insect biology and insect-pathogen interactions.99 Vector control strategies are now focusing on interference of arbovirus development in vectors and impacting insect survival, such as using enhancing vector immune system, manipulating vector microbiome, or editing vector genomes using clustered regularly interspaced short palindromic repeats-Cas systems.99 Despite the success of the old strategies and the prospects of the recent developments, failure of the vector control methods is still prominent in several countries; therefore, vaccination remains the most effective means of prevention.100
While vaccines may be the greatest way to prevent arboviral infections, vaccine hesitancy is a major barrier to infectious diseases like arboviral diseases around the world.100-102 Vaccine hesitancy has been impacting vaccine perception and acceptability in recent years.101 This could be due to some factors, including social media misinformation, mistrust of government or policymakers, religious convictions, and negative parenting.103,104 In some parts of the world, the emergence of anti-vaccine movements capable of aggressively influencing state legislatures has also been reported.36 Interestingly, vaccine hesitancy is significant in low- and middle-income nations, where many vaccine-preventable diseases continue to kill people every year, particularly children, who account for 1.5 million deaths.103,105-109 These countries include those with a high burden of arboviral diseases in recent times, such as Brazil, Indonesia, Mexico, India, Bangladesh, and Pakistan, with cases of severe morbidities and mortalities.110,111. This implies that the introduction of arboviral disease vaccines will suffer the same fate, limiting the influence of vaccination on arboviral disease incidence in those communities.103,112 As with other vaccine-preventable diseases, including measles, poliomyelitis, rubella, and mumps, vaccine-hesitant communities that remain under-vaccinated or non-vaccinated are susceptible to outbreaks of arboviral diseases.113 Furthermore, compared with other communities with high vaccination rates, vaccine-hesitant communities would have higher morbidity and fatality rates due to the unchanged prevalence of arboviral infections.114
CONCLUSION
Vaccine development would undoubtedly reduce the prevalence of arboviral infections and avoid epidemics in the future. Vaccines should, however, be highly efficacious, immunogenic, safe, and effective in all age groups, and offer long-term protection, as expected. Even though vaccine development has been successful thus far, the expanding geographic range of virus activity has undeniably increased the global burden of arboviral infections, as well as global case reports and the prevalence of severe cases. Even though vaccine development acceleration technologies are available, and have been proven to be safe and effective, there are still certain obstacles, such as funding and an unreachable target population. Bringing together global public health institutions, government agencies, pharmaceutical companies, and non-governmental organizations to work on vaccine research with a united purpose could be a definitive answer to such obstacles. On the other hand, rising vaccination hesitancy is another key obstacle to vaccine adoption, creating a substantial public health risk. Individual-level countermeasures based on behavioral and communication strategies, as well as community-level policies on vaccine requirements, are critical.