Abstract
Previous studies have demonstrated that the vision of one’s own body, or of external embodied limbs, can lead to pain relieving outcomes. Analgesic effects have also been related to the vision of illusory limb movements. Nonetheless, whether these two processes can be put together to obtain a summatory analgesic effect is not yet clear. The aim of this work was to investigate if it is possible to combine the analgesic effects of looking at one’s own body with those deriving from the illusion that one’s own limb is moving. Thirty-eight healthy participants underwent four visual conditions in immersive virtual reality while their heat pain thresholds were measured. In different conditions the subject watched from a first-person perspective: i) a still virtual arm, ii) a moving virtual arm, iii) a still non-corporeal object, and iv) a moving non-corporeal object. All participants were asked to keep their arms completely still during the visual exposures. After each condition, participants answered questions about their illusory experience. Our results show that the vision of the ‘own’ body significantly increased participants’ pain threshold as compared to the vision of the non-corporeal object. However, no statistically significant analgesic effect of vision of the virtual arm movement was found. The implications and limitations of this study are discussed.
INTRODUCTION
The relationship between pain perception and body-part movement is not new. Many studies have shown how strong the bidirectional relationship between the two can be.1 However, what is relatively new are studies relating limb pain and movement representation techniques such as mirror therapy, motor imagery, and movement observation.2,3 For instance, in the classic mirror therapy technique, patients with phantom limb pain move the healthy limb while watching its reflection on a mirror positioned in front of them, namely between the healthy limb and the residual limb. In this way, the patient has the illusion that the missing limb is back on its site and is fully functioning. The visual exposure to the illusory movement allows the reduction of the patient’s perceived pain.4,5 This is because of a modification of the body representation, which embodies the reflected limb, replacing the missing paralysed phantom one.6 At a neural level, studies making use of brain stimulation techniques have shown a link between pain states and motor neural networks, finding that a stimulation of the latter may positively translate into effective pain management.7-10 In one study combining transcranial direct current stimulation of the motor cortex and visual illusion on patients with spinal cord injuries, Soler et al.11 found that, despite transcranial direct current stimulation and visual illusion being both valid analgesic techniques, the combination of the two yielded the greatest and longest lasting pain relief. In a successive work, Villiger et al.12 found that the exposure to illusory own limb movements via a non-immersive virtual reality (VR)-augmented technique improved neuropathic pain in patients with incomplete spinal cord injury. Similarly, watching a video of hand movements led to a body site-specific increase of the pressure pain threshold in a group of healthy participants.13
Interestingly, not only the vision of limb movement, but also the vision of the own body has been shown to yield analgesic benefits. Although some studies failed to find such an effect, the majority of studies have found a reduction of pain at the sight of the own body that holds true even during the observation of prosthetic or virtual body parts provided that these were perceived as belonging to the participants’ own body.14
In support of this body-related visually induced analgesia, studies have pointed at an activation of the inhibitory GABAergic interneurons in the somatosensory areas in response to the vision of the own body.15-17 After all, a strong connection between internal body representation and pain conditions has been highlighted in several studies taking into account chronic pain patients that reported a distorted body image.18
VR is a tool that has been effectively used in neuro-rehabilitation and neuroscience, including pain management. For instance, it has been shown that VR can be effectively used as an adjunctive treatment in patients with severe burns, and that its effects are comparable to opioids.19-21 Interestingly, immersive VR (“a typical immersive VR system delivers stereo vision that is updated as a function of head tracking, possibly directional audio, and sometimes some type of limited haptic interface”)22 gives the possibility to display digital characters (avatars) that can be seen from a first-person perspective (1pp). In this way, it is possible to deceive the brain and challenge the user’s normal body representation, generating body ownership illusions (BOIs) over the avatar’s body.23 Based on these premises, in the current study we wanted to investigate whether the analgesic effects of vision of the own body could be added to the analgesic effect of the vision of illusory limb movement. To do so, participants were exposed to four different visual conditions in immersive VR, where either an avatar’s arm or a non-corporeal object (i.e. a purple tube) were displayed in place of their real arm. Both the avatar’s arm and the tube either performed a regular movement or kept still, while participants’ arms were always still. Importantly, in a previous experiment (unpublished data) we found that it is possible to induce BOI over a moving avatar’s arm seen from a 1pp, despite the visuo-motor mismatch between the participant’s still limb and the avatar’s moving limb. Increasing ramps of heat stimuli were applied to the participants’ wrists during the visual exposures. Our hypotheses were that participants’ pain thresholds would be higher during the conditions where the avatar’s arm was displayed, and highest during the vision of the moving avatar’s arm. We also expected to find stronger BOIs in the body conditions, which were likely to be highest during the vision of the still avatar’s arm (because of multisensory correspondence with the real arm). The strongest feeling of own arm movement illusion was predicted to be found in the avatar’s arm moving condition.
MATERIAL AND METHODS
Participants
Forty-two subjects were initially recruited for the experiment, a sample size based on previous similar studies.24-28 Four of these were discarded because their pain threshold was <40˚C,29 one because of an instructions breach, and another one because they were ambidextrous. Therefore, 36 healthy participants (20 females, mean±standard deviation [SD] age: 24.9±4.7 years, age range: 20–45) were included in the final analysis. They were all right-handed (mean±SD: 91.42±11.65, range: 62.5–100) according to the short version of the Edinburgh Handedness Inventory.30 This was important because stimuli induction and visual representation involved the use of the right arm; therefore, only right-handed participants were considered in order to rule out possible confounding factors due to laterality, and to increase homogeneity within the sample. They had normal or corrected-to-normal vision, no history of neurological disorders, and no other condition potentially interfering with pain sensitivity (e.g. drug intake). Upon arrival at the laboratory they were asked to read and sign a consent form. The experiment was approved by the local ethics committee and was in accordance with the Declaration of Helsinki.
Virtual Reality System
The stereoscopic head-mounted display (HMD) was an Oculus Rift DK2 (Oculus VR, Irvine, California, USA) with a resolution of 960×1080 per eye and a field of view of 100˚, displayed at 60 Hz. The virtual environment was programmed using the Unity platform (Unity Technologies, San Francisco, California, USA). Noise isolation was ensured by the administration of pink noise via headphones, with a constant volume set at 70 dB sound pressure level.
Thermal Stimulation
Thermal heat stimuli were delivered by means of a TSA-II Neuro Sensory Analyzer (Medoc Ltd., Ramat Yishai, Israel), with a 30×30 mm thermode tied with a Velcro® strap on the palmar side of the right wrist. The probe temperature was increased from normal skin temperature (constant baseline temperature=32°C) at 2°C/s. Participants were asked to press a button with their left hand as soon as they perceived the stimulation as being painful. Immediately after pushing the kill-switch button the temperature reached was recorded as the pain threshold and the probe temperature rapidly decreased to the baseline level (32°C). A fixed maximal temperature of 51°C was set for safety reasons.
Procedure
Upon arrival, participants were invited to sit on a chair and to read and sign the consent form. Then an experimenter secured the thermode on the participant’s right volar forearm close to the wrist, with a Velcro strap, ensuring the surface of the thermode remained in contact with the skin evenly. Before donning the HMD, participants were familiarised with the heat stimuli.
As the participants donned the HMD the room lights were turned off and the pink noise played. Regardless of the visual condition they were asked to visually explore the virtual scenario, and in particular to look down as if they were looking at their real body. All subjects went through the same four visual conditions (within subject design): i) a virtual body (avatar) seen from the 1pp, with its right arm still, ii) as in ‘i’ but the avatar’s right arm moved, iii) no virtual body, only a virtual tube in replacement of the virtual right arm, and iv) as in ‘iii’ but the tube moved (see Figure 1 for all visual conditions). The conditions differed on the movement because the virtual object could be still or in motion, and on the nature of the virtual object, which could be similar to a real human arm or a simple purple tube. All participants completed the four conditions, with the order of the conditions being balanced across participants. The movement consisted of a constant angular speed (ω) of 5.0, and the object moved 22.5° towards the right and 22.5° towards the left horizontally, using the elbow as a pivot.
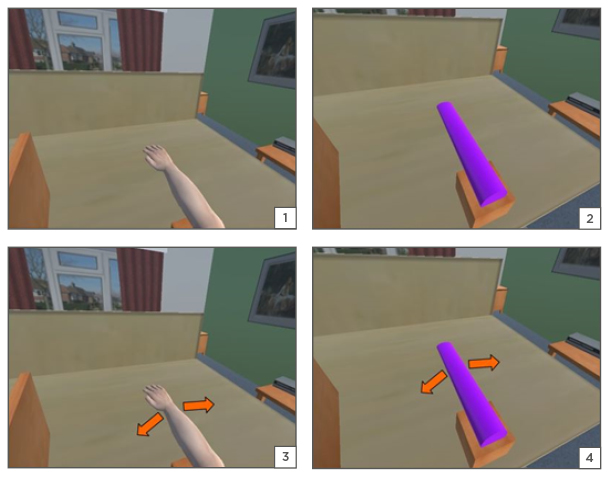
Figure 1: Screenshots of the experimental conditions.
From top left to bottom right: 1) arm still, 2) tube still, 3) arm move, 4) tube move.
To match participants’ physical features as much as possible, both the sex (male or female) and the avatar’s skin colour (black or white) was pre-selected. Care was taken to match the position of the real participant’s body to the virtual body as well as the real right arm and the right virtual arm/tube; the participants were asked to mimic the avatar’s body posture as much as possible, particularly for the right arm position, until they felt perfectly co-located with the virtual body. Participants were asked to keep their arms still during all VR conditions and to focus their attention on the right virtual arm/tube. Before the visualisation of the virtual scenario, participants were asked to lay their right elbow on top of a small box, rendered in VR below the avatar’s right elbow. This was done to limit the sensorial mismatch between the still real arm and the moving avatar’s arm/tube (in the movement conditions) to the movement itself, and to limit the expected tactile sensation that would have been produced by the avatar’s arm rubbing the table during the movement. To match the avatar’s arms position, participants’ lower arms were kept perpendicular to the shoulder-shoulder axis and at ˜20 cm distance from the torso.
During each visual condition four heat stimuli were provided, with an inter-stimulus interval of 30 seconds; since the avatar’s arm movement was kept constant and the end of the thermal stimulation was under the participants’ control, the recording of the specific avatar’s arm position by the time the heat stimulation was stopped was not possible, nor within the scope of this study. Each observational condition lasted for about 2 minutes. At the end of each condition, the thermal pain threshold was obtained by averaging the four participants’ thresholds. A total of 16 heat ramps were administered by the end of the experiment. After the fourth heat pain threshold had been recorded the visual condition terminated with the removal of the HMD and the subject responded to the questionnaire.
Subjective Measures
A questionnaire at the end of each experimental condition was administered to measure the subjective feelings experienced by the subjects during exposure to VR. Items were partly selected from a questionnaire used in seminal work on virtual arm ownership.31 The order of the items was randomised per each condition and participant. Importantly, before the administration of the questionnaires, participants were told that there were no right or wrong responses and that the only ‘right’ response was the one that corresponded to their feelings. This was done to ensure that the data reflected the genuine experience of the participants, hence limiting confounding factors such as compliance with ‘ideal’ expected responses. To ensure that each item was correctly interpreted, the questionnaire was read to the participant by the experimenter. Participants answered verbally using a 7-point Likert scale, with 1 meaning ‘totally disagree’ and 7 representing ‘totally agree’.
Items:
- Q1: During the experiment there were moments in which I had the sensation of having more than one right arm.
- Q2: During the experiment there were moments in which I felt as if the virtual arm/tube was my own arm.
- Q3: During the experiment there were moments in which I felt as if my real arm was becoming virtual.
- Q4: During the experiment there were moments in which the virtual arm/tube started to look like my own arm in some aspects.
- Q5: During the experiment there were moments in which I had the sensation that the heat was coming from the virtual arm/tube.
- Q6: My attention was totally focussed on other things, for example, in what I was watching1 or totally on the thermal stimulus.7
- Q7: During the experiment there were moments in which it seemed that my real arm was moving.
Data Handling
Single trial pain thresholds (in °C) were averaged for each visual condition and subject. Given the high variability between participants, a check of the outliers was carried out. Out of 144 values, 19 values were identified as outliers (>1.5-times the SD from the group’s mean) and replaced with the mean scores of the group for the same visual condition.
Resulting data from all conditions were normally distributed according to the Jarque–Bera and the Shapiro–Wilk tests32 (all ps>0.05). A 2×2 repeated-measures ANOVA (two factors: ‘Body’ and ‘Movement’, both with two levels) was then conducted on mean pain thresholds. The level of significance was set at p<0.05. Questionnaire scores were averaged across subjects per each item and visual condition. The resulting mean scores related to each question were subjected to Friedman ANOVAs. Post-hoc analysis was carried out with Wilcoxon Matched Pairs Tests, with a Bonferroni correction applied for the number of possible comparisons. These comparisons were carried out between ‘arm still’ versus ‘arm move’, ‘arm still’ versus ‘tube still’, ‘arm move’ versus ‘tube move’, and ‘tube still’ versus ‘tube move’. This resulted in a significance level set at p<0.0125.
RESULTS
Pain Threshold
The one-way repeated-measures ANOVA revealed a main effect of the factor ‘body’ (F1,35=5.30, p=0.027, Partial Eta squared=0.13) so that irrespective of the presence of movement, the vision of the avatar’s arm was linked to a significantly higher pain threshold as compared with the vision of the object (Figure 2). No main effect of the factor ‘movement’, nor an interaction between the two factors ‘body’ and ‘movement’ were found to be significant (respectively: F1,35=0.008 and F1,35=0.88, all ps>0.05). So, our first hypothesis that there would be a higher pain threshold during the vision of the avatar’s arm was confirmed, while the second hypothesis that the highest pain threshold would be during the vision of the avatar’s arm movement was not statistically supported.
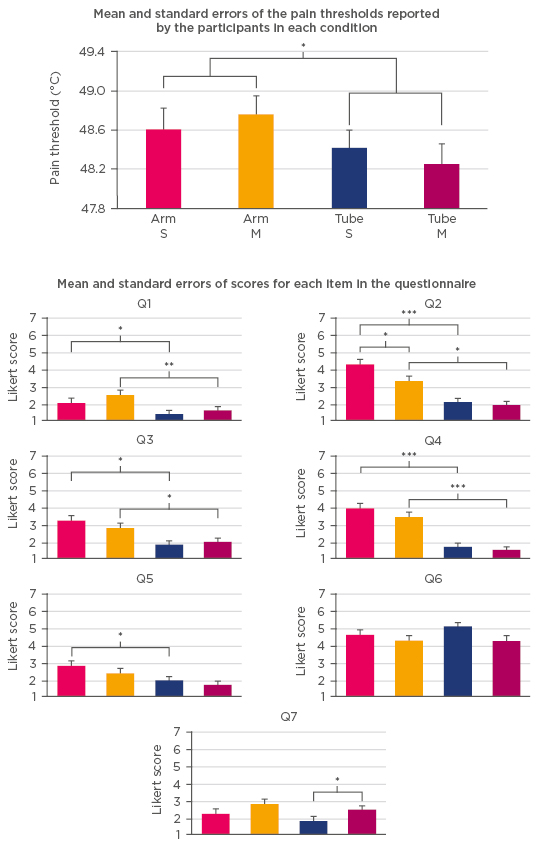
Figure 2: Top row: mean and standard errors of the pain thresholds reported by the participants in each condition (from the left: ‘Arm S’, ‘Arm M’, ‘Tube S’, ‘Tube M’). Centre and bottom: mean and standard errors of scores reported at each item of the questionnaire. Colours match the same conditions as in the ones depicted in the pain plot. Only significant contrasts are denoted.
*p<0.05, **p<0.01, ***p<0.001 (Bonferroni p-corrected level=0.0125).
Arm S: arm still; Arm M: arm move; Tube S: tube still; Tube M: tube move.
Embodiment Scores
Analysis using Friedman ANOVA of Q1 scores reported a significant p-level (χ23=27.06, p=0.00001). The sensation of having more than one right arm reported in the ‘arm still’ condition was significantly higher compared with the ‘tube still’ condition (Wilcoxon post-hoc test, p=0.007) and that in the ‘arm move’ versus the ‘tube move’ condition (p=0.0003), despite the mean values from all conditions being very low.
On Q2 scores, the analysis with Friedman ANOVA showed a significant p-level (χ23=44.78, p<0.0001). As hypothesised, the sensation of ownership towards the virtual corporeal or non-corporeal object reported in the ‘arm still’ condition was significantly higher than in the ‘arm move’ condition (Wilcoxon post-hoc test, p=0.0018) and also higher than the one in the ‘tube still’ condition (p=0.00004). Furthermore, the sensation of ownership reported in the ‘arm move’ condition was significantly higher than the one in the ‘tube move’ condition (p=0.00084).
The analysis with Friedman ANOVA on Q3 scores reported a significant p-level (χ23=30.50, p<0.00001). The illusion that the real arm was becoming virtual was significantly stronger in the ‘arm still’ condition compared with the ‘tube still’ one (Wilcoxon post-hoc test, p=0.001), and it was also significantly stronger in the ‘arm move’ condition compared with the ‘tube move’ condition (p=0.003).
Q4 scores, analysed with Friedman ANOVA, showed a significant p-level (χ23=64.14, p<0.00001). As expected, the sensation of similarity between the real arm and the virtual object reported in the ‘arm still’ condition was higher than the one reported in the ‘tube still’ condition (Wilcoxon post-hoc test, p<0.00001), and the same pattern is reported between the ‘arm move’ condition versus the ‘tube move’ (p=0.00001).
Analysis using Friedman ANOVA on Q5 scores reported a significant p-level (χ23=20.59, p=0.0001). In particular, the sensation that the heat was coming from the virtual arm/tube was stronger in the ‘arm still’ condition compared with the ‘tube still’ (Wilcoxon post-hoc test, p=0.0026). The analysis with Friedman ANOVA on Q6 scores failed to report a significant p-level (χ23=7.7, p>0.05). That meant that no significant differences were found in terms of attentional resources drawn by the different conditions.
Lastly, the analysis with Friedman ANOVA on Q7 scores reported a significant p-level (χ23=13.75, p=0.003). A slightly stronger sensation of own arm movement was found in the ‘tube move’ condition as compared with the ‘tube still’ condition (p=0.008). Thus, our original prediction that the highest arm movement illusion would be during the vision of the avatar’s arm was not statistically supported. No other comparison was found to be significant.
DISCUSSION
The present study aimed at investigating whether it is possible to boost analgesia through the combination of the vision of the body and limb movement observation. However, with our set-up, we did not find a statistically relevant higher pain threshold during the vision of the avatar’s arm movement. What we found is a main effect of the vision of the body, in line with previous studies reporting an analgesic effect by looking either at the real or the dummy body.33-35 At a subjective level, we found that the conditions where the arm was displayed reported significantly stronger levels of BOI (Q2) compared to the tube ones. If this result could be, on one hand, expected, on the other hand it appears less obvious when we consider that the tube, like the avatar’s arm, was co-located with the real arm, and that even in the condition when the avatar’s arm is moving the BOI was significantly stronger compared to the tube one. One could argue that the difference in pain threshold reported by this study could be due to a stronger capturing of participants’ attention by the arm conditions compared to the tube conditions. Indeed, attention is one of the most important pain modulators,36,37 and VR-based distraction has been effectively used to reduce the pain felt by patients with severe burns undergoing clinical treatments.20,21 However, we did not find any significant difference between conditions in terms of attentional resources drawn, in fact the arm and tube conditions followed very similar trends on Q6. Hence, the variation in thermal pain thresholds may be because of the subjective experience related to BOI. In fact, overall, we found a significant difference between the arm conditions and the tube ones in the first four questions. Under BOI paradigms the illusion of having more than one right arm (Q1), the illusion that the real arm is becoming virtual/rubber (Q2), and that the virtual/rubber arm resembles the real one (Q3) are usually considered as control questions, but recently a relation between these illusory feelings and high levels of BOI has been found in some studies.38-40
Nonetheless, we failed at finding a significant effect of the movement factor on pain threshold, either alone or in combination with the body factor. This may be because the avatar’s arm movement can be considered as an asynchronous feature, and previous studies demonstrated how synchronous and asynchronous conditions can affect the level of BOI.25,40 So, as shown by the subjective reports for the item on illusory movement (Q7), the feeling that “…my real arm was moving” is likely dampened by the multisensorial mismatch. In any case, although not statistically relevant, we still observed a clear trend in which the arm movement condition reported the highest pain threshold, even higher than in the condition where the avatar’s arm is still; that is where the visual and the proprioceptive inputs are coherent. Recently it has been shown how the spatial correspondence between the real and the virtual arm is key to make the body-related visually induced analgesia stand out.41 In the present work, we found that despite the continuous visuo-spatial mismatch, the arm moving condition reported a slightly higher pain threshold compared to the arm still condition. Thus, despite the non-significant effect of movement, results are encouraging and future studies may want to further investigate the role of illusory own limb movement with slightly different experimental designs and/or set-ups. For instance, future works could investigate if different features of limb movement, such as speed, amplitude, or type of movement, may induce higher level of body ownership and of kinaesthetic illusion in the participants, to see whether and how this translates into pain modulation.
We are aware that the current study presents some limitations: for instance, the evaluation of the body ownership via self-reported questionnaire could have been accompanied by more objective measures, such as galvanic skin responses to a threat menacing the virtual arm. Also, a more realistic virtual arm could have helped improve the BOI during the avatar’s arm conditions. Yet, it is notable that our results show that it is possible to generate an illusory sense of ownership over a moving virtual arm despite a strong visuo-motor mismatch between vision and proprioception. This may have therapeutic implications targeting patients with limb movement disorders or immobilised limbs.