Abstract
There is a growing need for personalised medicine to manage and prevent diseases, as well as to monitor patient health continuously, even outside the hospital setting. Biosensor devices hold a huge potential in addressing this need and can aid in decentralising healthcare by offering faster disease diagnosis and prognosis. Integrating biosensors with wearables such as contact lenses has widened their scope of application and addresses some key challenges associated with other techniques, such as invasive monitoring and the need for bulky equipment. Indeed, traditional approaches tend to be invasive, such as those used for diabetes management that require regular finger pricks to obtain a blood sample to monitor glucose. In contrast, smart contact lenses use tears, which are naturally and regularly replenished. Tears are rich in biomarkers such as proteins and electrolytes, and variations in their concentration can be monitored non-invasively to offer valuable information related to the onset or progression of disease. In addition, intraocular pressure monitoring for glaucoma has been achieved using contact lenses utilising nanomaterials and microfluidics technology. With these promising successes, current researchers seek to address neurodegenerative diseases and cancer diagnosis and prognosis using contact lenses. Contact lenses can also be used as ocular drug delivery systems as they possess 10 times more drug bioavailability advantage than topical administration. By combining contact lenses with a sensing platform, a power source, an antenna, a data transmitter, and a display, smart contact lenses have become more than vision collection devices. Although considerable challenges remain to be tackled because we are still at the initial stages of this technological advancement, the number of diseases and drugs that could potentially be sensed and delivered, respectively, seems vast.
Key Points
1. Utilisation of smart contact lenses (SCL) for wearable biosensors and drug delivery can have a significant impact on personalised medicine. This paper reviews the current progress and uses of SCLs in healthcare, in combination with their challenges such as the need for non-invasive, continuous monitoring, and efficient drug delivery, potentially transforming disease management and prevention.
2. This review describes the technological innovations in the field of SCLs. The biomarkers that are present in tears and particular diagnostic, therapeutic, and prognostic applications are explained. The challenges and limitations that need to be addressed for the realisation of the full potential of SCLs in wearable biosensors and drug delivery are outlined.
3. There have been promising developments and technological innovations that reveal the strong potential of SCLs for medical applications. Overcoming the existing challenges could transform healthcare by decentralising it, making it more accessible and efficient towards precision medicine.
Introduction
The global market of contact lenses (CL) has been significantly growing in recent years. Contact lenses are worn for vision correction or cosmetic reasons, making them ideal candidates for non-invasive applications in monitoring biomarkers in tears for the diagnosis of ocular and neurodegenerative diseases.1 CLs have also been used in the medical field for drug delivery in disease treatment, besides other non-medical applications such as eye tracking during virtual reality gaming and as displays for augmented reality.2 CLs are placed on the eye’s surface in close contact with ocular fluids like tears, aqueous, and vitreous humour rich in biochemical analytes.3 The tear film provides the eye antibacterial properties, oxygen transfer, corneal lubrication and protection, nutrition, and washes debris out. It consists of three layers: the lipid, watery, and mucin (Figure 1). Comparative studies between analyte concentrations in tears and blood have been carried out during the last decade, with the results indicating a positive correlation.4 Analytes in tears such as glucose, lactate, urea, and primary ions of electrolytes Na+, Ca2+, K+, Mg2+, and Cl– have shown a correlation with the equivalent blood levels.4 Moreover, over 50 different proteins have been detected in tears and continue to be investigated as possible biomarkers for additional disease diagnostics such as cancers and renal functioning.5
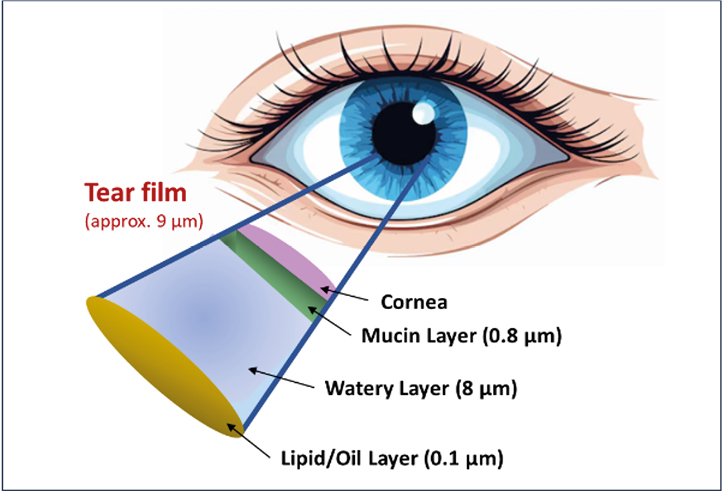
Figure 1: Structure of the tear film
A contact lens is a thin, curved layer made from a transparent or slightly tinted soft or rigid material worn in direct contact with the cornea. CLs are classified according to the materials used and are either soft, rigid, or hybrid. Soft materials used include hydroxyethyl methacrylate (HEMA), polyacrylamide, polyethylene terephthalate, and polyvinyl alcohol;1 rigid materials are polymers like polymethyl methacrylate; whereas hybrid CLs are a combination of soft and rigid materials as illustrated in Figure 2. Integration of microcircuits, microsensors, and other technologies like microfluidics, data transmission, micropower supply systems, and display circuits with a contact lens has led to the development of ‘smart contact lenses’ (SCL). Poly-HEMA hydrogels or silicones are the most popular materials used in SCLs for biomedical applications.6
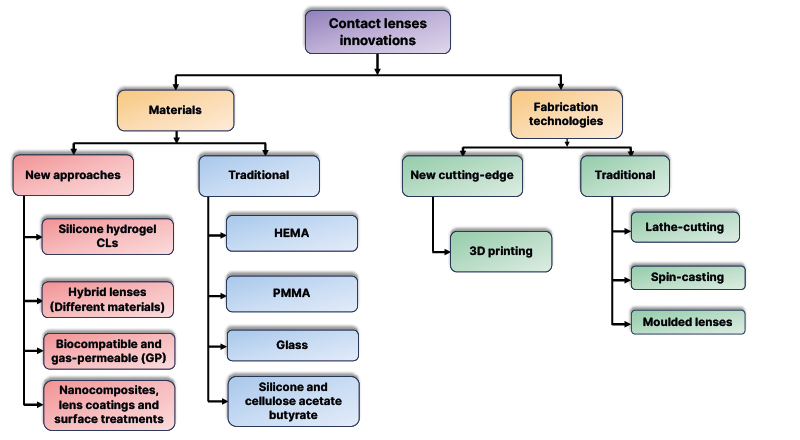
Figure 2: Recent innovations in contact lenses in terms of materials and technology employed
The proximity of contact lenses within the tear film offers some advantages for real-time monitoring applications. This is made possible because of the continuous replenishing of tears, which contains a wealth of information about our health. CLs offer a minimally invasive option for disease monitoring compared to other options like finger pricking for blood samples.7 Alternative sources of analytes include sweat, saliva, and interstitial fluid (ISF); however, cross-contamination, for instance, in the mouth may compromise the accuracy of the results for saliva.8 Skin irritation for smart patches in ISF applications has also been reported, pushing the frontiers of research towards tear-based biosensing.9, 10
Advances in materials science have enabled the use of nanomaterials in biosensing to detect changes at the nanoscale level and develop miniaturised transducers. These innovations may help overcome the analytical challenges of detecting low concentrations of certain molecules in tears in the future.11,12
BIOMARKERS IN TEARS
Biomarkers play a critical role in disease diagnosis, prognosis, and prediction of response to therapy. They offer essential insights into disease progression and can inform on appropriate therapeutic approaches. For a long time, biomarkers have been explored invasively through blood sample collections, although recently, tears have received attention as an alternative biomarker source. The two techniques widely used in tear sample collection are Schirmer strips and capillary tubes. The results have indicated that the concentration and composition of biomarkers often depend on the sample collection method, the tear type, and the post-processing handling procedures.13 Nonetheless, new biomarkers have been discovered for diagnostics beyond ophthalmic conditions to include neurodegenerative diseases like Parkinson’s disease, Down’s syndrome, Alzheimer’s, and dementia.14,15
Some key biomarkers that have been measured by electrochemical biosensors include glucose for diabetes, creatinine for renal function, dopamine for glaucoma, lactoferrin for keratoconus, and cortisol for neurological diseases.16 Electrochemical-based transduction techniques are preferred for tear-based diagnostics9 as optical methods are hard to accurately quantify the concentration other than being an indicator that an analyte is present above a certain threshold.7 Tears can also be used for cancer diagnosis. Some examples of potential tear-based biomarkers include lacryglobin for colon, lung, prostate, and ovarian cancer; cystatin SA, miR-21, and miR-200c for breast cancer; and lactoferrin and cystatin C for eye tumours.17 Further investigations continue to be undertaken to identify more selective and specific biomarkers for diagnosis and early detection of other human diseases.
DIAGNOSTIC APPLICATIONS
Disease diagnosis is very important in healthcare as it provides information on the patient’s condition and informs on decisions taken to manage it. Diagnosis has always been carried out in centralised labs in hospitals and often requires complex sample preparation procedures and bulky equipment. Biosensors have enabled faster and highly sensitive alternatives. SCLs have been and continue to be investigated as potential commercial products for disease diagnosis and as drug delivery microsystems.
Diabetes
One of the diseases that has received a lot of diagnostic attention in using SCL sensing is diabetes. Increased glycaemic levels in the blood due to uncontrolled glucose metabolism results in diabetes and increases the risk of cardiovascular disease development. Other associated conditions such as diabetic retinopathy, diabetic nephropathy, and diabetic neuropathy can develop, potentially leading to life-threatening consequences.18 This has necessitated point-of-care and self-testing glucose monitoring for effective diabetes management. A downside of blood glucose monitors is the need for a finger prick to obtain a blood sample.18
In 2014, Google (Mountain View, California, USA), in partnership with Novartis (Basel, Switzerland), started testing SCLs that were built to measure glucose levels in tears for potential use in diabetes management.19 However, the project was halted in November 2018. The developed prototype had an electrochemical sensing component, a power supply, an antenna, and a light-emitting diode encapsulated between two lens materials.5 Besides miniaturisation challenges, there have been concerns about the real-time correlation of glucose levels in tears compared to glucose levels in blood due to lag time in tear measurements, and this was the primary reason for the Google Lens project halt. However, after compensation for the lag time, a positive correlation was observed, indicating the possibility of still using SCLs for the diagnosis and monitoring of diabetes and insulin intervention.20,21
Electrochemical transduction has been the most used technique in SCLs for glucose monitoring using enzymes such as glucose oxidase and glucose dehydrogenase, and lactate oxidase for lactate.22 Concentrations of glucose in the range of 0.01–20 mM have been reported in the literature.23 Optical methods based on nanoparticles embedded in contact lenses have also been explored, with reported linear regression values in the range of 0.1–0.6 mM.18
Glaucoma
Glaucoma is an irreversible eye disorder that results from increased intraocular pressure (IOP), which affects the optic nerve and can lead to loss of vision.24 IOP is the equilibrium between the production and outflow of aqueous humour. There are two types of glaucoma, with open-angle glaucoma being the most common condition, and it results from an ineffective drainage angle in the eye hampering the outflow of aqueous humour.24 This leads to progressive elevation of IOP, which can lead to destruction of the optic nerve. The angle-closure glaucoma is not common but is often abrupt and severe and requires immediate medical attention. It occurs when the drainage angle of the eye gets blocked, leading to a sudden surge in IOP.24
Clinical IOP measurements use the Goldmann applanation tonometer, which is somewhat invasive since the device comes into close interaction with the cornea, requires an anaesthetic application, and needs to be handled by a trained professional.25 Advances in technologies such as microfluidics and biosensors are changing IOP sensing using contact lenses and can offer a continuous measurement application that promises a better understanding, taking into account that IOP varies throughout the day and depends on activity levels. The changes in the volume of liquid in microfluidic channels induced by pressure changes in the eye are monitored as an indicator of IOP.12 Compression on a holding chamber with liquid pushes the liquid into the microfluidic channel where the volume reading is taken using an external image processor such as a digital or smartphone camera.6
Further advances using nanomaterials to monitor IOP have been reported. In 2021, a prototype SCL was developed using a transparent silver nanowire strain sensor with wireless power and communication capability.26 In vivo trials of the SCL on living rabbit eyes, validated by tonometer readings, indicated the feasibility of use in monitoring IOP of patients with glaucoma.26 Sensimed AG developed the SENSIMED Triggerfish® (Sensimed, Etagnières, Switzerland) SCL for IOP monitoring and glaucoma treatment. It is one of the companies that have received FDA approval for its SCL to be commercially used in vivo for IOP measurements,27 demonstrating viability in wider applications.
Stress
Human cortisol is a steroid hormone produced by the adrenal glands that has received significant attention as a biomarker for stress. It is excessively secreted as a response to stress and is associated with depression and anxiety.24 Immunoassay and chromatographic approaches that are used in cortisol monitoring require bulky equipment. This has necessitated the development of wearables such as soft SCLs for real-time monitoring of cortisol in tears. A SCL with a cortisol sensor, microcircuits, antenna, and capacitors was developed using a graphene-effect transistor to produce a measurable electrical signal and achieved a limit of detection of 10 pg/mL.27 Cortisol concentration in tears is about 82.76 ng/mL and increases as stress levels rise.27 Despite higher levels in blood, serum, and urine, tears offer the advantage of continuous monitoring and non-invasiveness. Electrochemical methods have shown potential in cortisol sensing with antibodies, enzymes, and molecular imprinted polymers (MIP) used as receptors, and attempts have been made for their integration into wearables like CLs.28 However, most of these explorations have been focused on human sweat and saliva among the less invasive options regardless of the enormous potential of CLs.28-30
For instance, a sensing system using photonic hydrogel was developed exploiting MIP and antibody–antigen competitive binding and tested with sweat, while another was based on a fluorophore and aptamer competitive assay to detect cortisol.31 These principles can potentially be explored in SCLs for cortisol detection and monitoring.31,32 Given that long-term exposure to stress can trigger anxiety, depression, and cardiovascular diseases, and potentially lead to drug and substance abuse,33 monitoring and studying stress is very important. Biosensing using SCLs offers a platform for achieving this endeavour as prognostic and diagnostic tools.33
THERAPEUTIC APPLICATIONS
SCLs have been used in glucose monitoring but have also been explored for drug delivery in treating diabetic retinopathy, which is responsible for most adult blindness.1 Current treatment methods for diabetic retinopathy are highly invasive, and they either involve laser irradiation, eye injection, or surgery.16,21 However, SCLs have demonstrated the ability to release embedded therapeutic drugs at the trigger of diabetic retinopathy symptoms.6 Current research explores the possibilities of expanding the number of drugs that can be administered using SCLs. For instance, metformin used for the treatment of diabetes is commercialised as an oral drug, but ocular administration with the use of SCLs is considered for its delivery.34
CLs can also be used as drug delivery channels in the management of other ocular diseases. Most of these diseases require topical administration, with eye drops being the most common way to deliver drugs through the cornea, although ocular barriers limit the drug bioavailability to less than 5% because of the short residence time in the tear film.35 CLs entrap the medication during polymerisation or by soaking the soft lens hydrogels in the drug, and this approach increases the drug bioavailability to about 50%.35 Johnson & Johnson’s drug delivery Acuvue Theravision CL (Johnson & Johnson, New Brunswick, New Jersey, USA) received FDA approval in 2021 as a daily disposable lens for delivery of H1-antihistamine for the prevention of itch in the eyes due to allergies.35
In other cases, CLs have been explored in the treatment of dry eye disease (DED). DED is the most common ocular disease, which occurs when there is insufficient lubrication of the eyes and causes damage to the eye tissues, which may lead to loss of vision.36 CLs loaded with cyclosporin A for the treatment of DED have shown impressive outcomes, stretching the possibilities of integrating SCLs with therapeutics.36 Perhaps more drug delivery using CLs in the treatment of DED will be investigated in the future, given most of the studies are at the clinical trial stage. However, the first treatment of DED is the application of artificial tears to increase the wettability of the eyes, and hydrogel CLs, have shown potential application in the hydration of dry eyes by altering the viscosity of the hydrating solution and entrapping it in the CL to increase retention time on the surface of the eye.36,37
PROGNOSTIC APPLICATIONS
Prognosis is the likelihood of a certain condition’s occurrence or disease progression following its detection or onset. Often, prognostic models are used to utilise multivariable characteristics of individuals to help clinicians make informed decisions on the treatment course for patients. However, one profound challenge is prognostic uncertainty, where there is inadequate data or history about a patient and a lack of model validation.38 Smart biosensing CLs in real-time healthcare applications collect crucial data during diagnosis and monitoring of disease progression, which is important for prognosis and development of prognostic models. The data collected can be utilised in potential prognostic applications as seen in Figure 3.
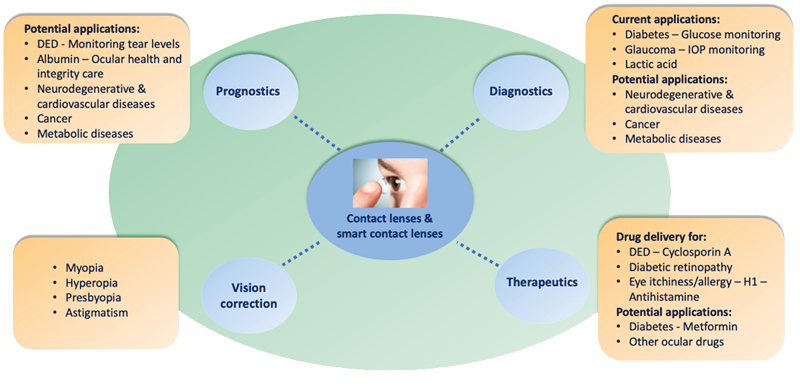
Figure 3: Medical applications based on contact lenses
Lately, healthcare management approaches have changed from reactive to predictive, preventive, and personalised, triggering the need for cost-effective devices and non-invasive biomaterials with minimal patient risks of complications. Traditional analytical techniques such as ELISA, mass spectroscopy, and electrophoresis assays require bulky equipment installed centrally and are time-consuming, often requiring invasive sample collection and extensive sample preparation procedures.39 Contact lenses and tears bypass most of the challenges posed by traditional approaches and provide an avenue for reliable options of prognosis outside the hospital environment. Clinical efforts have been made to identify and systematically categorise patients sharing the same risk of disease, such as cardiovascular disease, as a strategy formulation for preventive and predictive care.40 Identification of biomarkers for such diseases in tears can aid in the development of SCLs for data collection and monitoring in preventive care.
At present, some protein biomarkers like albumin can function as non-specific biomarkers, yet they offer insights into one’s ocular integrity. When high serum albumin is detected in tears, it is an indicator of plasma leakage and denotes issues with vascular permeability.41 Although the available data concerning albumin in tears is poorly understood, it has been demonstrated that wounded eyes and those with ocular disorders exhibit higher albumin levels.41 Monitoring tear albumin can thus provide information that can help understand its role, help in assessing the general ocular health of an individual, and trigger some specific disease diagnosis.42 In DED prognosis, contact lenses could be used to monitor tear levels in the eyes as a pointer to the disease’s commencement or progression.
CHALLENGES AND LIMITATIONS
Despite the tremendous efforts to accelerate the adoption of SCLs in diagnosis, prognosis, and drug delivery in healthcare, there are still several hurdles that need to be overcome before SCLs can be fully commercialised. The challenges associated with SCLs largely touch on the engineering materials and effective usability aspects. In terms of size, SCLs present miniaturisation challenges given the integration requirements of the wireless communication, power source, antenna, and sensing platform in such a small size as a contact lens.43 In addition, SCLs operate in the tear environment, which is ever-evolving and is posed with biochemical changes that may affect the attainment of clinical-grade results.44 Safety evaluation of SCLs for clinical application is lengthy, costly, and challenging given that they come into contact with the human mucosal membrane of the eye. They require animal and cytotoxicity tests, irritation, sensitivity, and implantation effect studies, which limits laboratory to commercialisation translation. Shelf life and technology limitations are other challenges that need to be overcome to achieve more clinical applications.45
The eye architecture presents design challenges whereby the SCL needs to be lightweight, maintain its transparency to serve its function, be comfortable and aesthetic to the wearer, discreet and unnoticeable to others, and limit any risks that may arise from contaminations.1 High transparency is crucial for daily use of the SCL, and a transparency rate of over 91% should be achieved, allowing for clear, natural, and unobstructed vision.46 In addition, this contributes to the integration of multiple biosensors without compromising the primary function of the contact lens, which is the provision of clear vision.1 Therefore, material selection is highly important for ensuring inherent high transparency and ensuring that the SCL does not obstruct vision; such materials are graphene and metal nanowires, and ultrathin film technology is widely used.1,47 In continuous applications, energy efficiency is paramount because the data transmission at regular intervals, the transduction mechanism that senses the biomarker-analyte interaction, and the data processing, require a lot of energy. Biofouling, which is the accumulation of foreign molecules on the surface of the sensing platform due to nonspecific binding, affects the reliability of the sensor.46 Sensor selectivity towards the analyte is another issue that must be addressed because of interference from other similar biological molecules.8
Biomarker concentration in tears poses detection challenges. Some biomarkers are present in minute concentrations and require highly sensitive and effective detection techniques. Some biomarkers have been fronted for multiple disease detection, which makes it difficult to assign them to a specific diagnosis.48 In therapeutics, challenges have been observed with the quick release of drugs from contact lenses, and in methods that use MIPs, the stiff cross-linking of the monomer chains affects the drug release.35 Also, the effects of drug encapsulation on the contact lens on properties such as oxygen permeability and wettability need to be understood.
Finally, a thorough and multidisciplinary investigation is required regarding the importance of future longitudinal studies to assess the effectiveness of SCLs in real-world biosensors and drug delivery applications.49 Some of these challenges include biosensor’s stability and function over extended periods of wearing, avoidance of interference from other substances in tears, long-term biocompatibility of the used materials, and the integration of the biosensor into the contact lens should not compromise its transparency, flexibility, and comfort.24,49 Advanced micro-manufacturing techniques, a reliable power source without adding bulk or weight to the contact lens, and practical and user-friendly methods for reading the data are essential, including wireless communication technologies that can transmit data to external devices without causing discomfort or inconvenience to the wearer.49
PROSPECTS AND INNOVATIONS
The global market for SCLs was valued at 115 million USD in 2018, and is projected to reach 1,603.4 million USD by 2026, with a significant compound annual growth rate of 38.9%.24 Many opportunities are presented by the emerging concept of SCLs, especially in diagnosing and monitoring different diseases. There is a need for the integration of multiple sensing platforms in one contact lens. For example, SCLs can be developed for both glycaemic control and IOP measurement.3 Initial human trials of glucose monitoring with SCLs have reported results that correlate glucose concentration in blood and tears, but they need further investigation.20 Other aspects that need exploration include therapeutic integration into SCLs, whereby, besides monitoring the variation in biomarker concentration, the drugs encapsulated in the lens can be automatically triggered and released for treatment of ocular diseases.
In terms of therapeutic applications, some ocular drugs that are poorly absorbed through the ocular membranes can be formulated into nanomicelles and nanosuspensions that can be delivered using CLs. By integrating smart sensing, these drugs can be dispersed when required by utilising, for instance, pH or analyte concentration changes.50
More research needs to be undertaken to identify and validate specific biomarkers in tears that can be used in the diagnosis of different diseases. Catecholamines can be studied for the diagnosis of stress and neurodegenerative diseases,51 and more biomarkers need to be identified for the detection of the different types of cancers. This can potentially enable the development of SCLs for those applications. Nanomaterials have also opened a new arena to address the challenges of biomarker concentrations in tears. By using nanomaterials together with other biological analytes, the sensitivity and selectivity of the sensor can be improved, making it possible for measuring concentration in the nano and picomolar ranges.52 To address the challenge of energy supply, effective wireless charging of SCLs can become feasible through wireless powering using radio frequencies in a 3D range or energy harvesting from the human body.24,43,53
CONCLUSION
SCLs have become an integral part of wearable devices, thus revolutionising disease diagnosis, prognosis, and therapeutics. As progress is being made in technological developments, so are advancements with smart wearables, including SCLs, thus addressing some current challenges with energy, miniaturisation, design, and fabrications. Research in microfluidics, materials, and pathology is opening room for the identification of more biomarkers in tears, widening the scope of diseases that can be diagnosed, and providing subsequent alternatives for drug delivery in ocular applications. In addition, adverse effects of SCLs should be significantly considered based on immunological, physiological, and mechanical factors, particularly during the selection of the materials. The innovation potential in SCLs is immense, especially in point-of-care diagnostics, personalised, and preventive healthcare management, and supporting the move towards attaining the highest level of healthcare quality. Nevertheless, we are still in the early stages of these innovations, and many challenges must be overcome before the adoption of SCLs as part of clinical techniques for quick and non-invasive diagnosis. Heightened focus should be aimed at bridging the gap between the laboratory, clinical trials, and commercially viable products for clinical applications. Overall, SCLs and wearable devices hasten the benefits that can be harnessed from the successful deployment of SCLs in not just vision correction and aesthetics but also important sensing platforms in healthcare management.