Abstract
Ultrasonography is often the preferred method of imaging for needle-guided procedures of the hip joint, including aspirations, local anaesthetic injections, and the use of corticosteroids. Phantoms (i.e., training devices mimicking human tissue and bony structures under acoustic sonography) are used to help train medical professionals to perform these procedures accurately, emphasising the art of physical simulation in unison with didactic learning. In the authors’ study, 3D printing technology was used to simulate an anatomically accurate human hip joint, with the hopes of creating an affordable and customisable ultrasound hip trainer, allowing novice sonographers to practice guided injections to hone their skills before performing these procedures on human subjects.
Key Points
1. Hands-on learning for ultrasound (US)-guided procedures is of increasing importance, as these procedures are growing within the field of medicine.
2. Tactile feedback trainers are important tools in US training. 3D printing technology can recreate sonographically scannable structures to create simulators at a fraction of the current market price.
3. This study investigated the use of in-house 3D printing technology and ballistics gelatine as cost-effective building materials for US phantoms. The model built was anatomically and sonographically comparable to a human hip joint, but cost <50 USD.
INTRODUCTION
As the use of ultrasonography rises within the medical field as a safe and effective way to diagnose and perform bedside procedures, the need for accurate, customisable ultrasound (US) trainers in the academic setting have become all the more apparent. In addition to teaching vital anatomical landmarks and US techniques, US trainers (phantoms) provide a realistic, hands-on experience for procedural practice for students and clinicians alike. Use of simulation-based learning is vital to long-term procedural skill and acuity; a notion that is further defined by the Best Evidence Medical and Health Professional Education (BEME) systematic review, where the first three main elements for ensuring effective procedural learning include active feedback during simulation, repetitive practice on a simulator, and integration of simulators within an overall curriculum.1 While the above emphasises the overall benefit of medical simulation at large, studies have since been conducted in order to highlight the benefit of simulation training within sonographic education specifically. In a literature review spanning over 19 studies, US simulation with task trainers not only showed an improvement in student confidence but had superior learning outcomes when compared with theoretical teaching strategies alone.2
The necessity of realistic simulation in conjunction with clinical experience throughout medical education is evident. This is especially true in case of hospital-based learning, as practitioner training is often a by-product of the facility’s main objective: patient care and outcomes.3 When combined with a standardised procedural curriculum, the use of scanning trainers in medical education has been shown to significantly improve student knowledge base, while also allowing additional practice time to better build procedural skill and precision.4
This need for further training and experience in US technique is applicable in the case of musculoskeletal scans of the hip joint, as musculoskeletal US guidance is a vital component of various procedures, including hip aspirations, local anaesthetic injections, and the use of hyaluronic acid or corticosteroids within the synovial hip joint.5 Due to the hip joint’s deep lying stature, lack of obviously palpable landmarks, and highly vascularised and innervated nature, procedures involving penetration of the pelvic girdle and associated attachments often require real-time imaging guidance.6 While US and fluoroscopy are both viable imaging modalities for these procedures, musculoskeletal US guidance is preferred clinically due to its timeliness, portability into the patient room, lower procedural cost, and lack of ionising radiation.7,8 While the utilisation of US phantoms in training for medical procedures has been shown to increase skill and accuracy, the use of these learning tools is often outweighed by cost. To date, the current market for medical educational tools displays a majority of cost-prohibitive phantoms with non-modifiable, non-reusable characteristics.
In response to the current US phantom market, this study sought to use 3D printing techniques, in addition to the authors’ prior years of experience making phantoms, to create an inexpensive and versatile hip phantom embedded into a biodegradable ballistics gelatine (gel). The authors’ 3D printed hip joint is created from an anatomically accurate model that was freely available online and maintains the anatomical relationships of the bones within the human hip, while the sustainable ballistics gel allows for texture and US appearance close to that of human flesh. The duality of this hip trainer, both as an US practice device for identifying key anatomical landmarks and a needle-accepting device for practicing hip-based US-guided procedures, makes the authors’ model a good alternative to other hip phantoms and training devices.
MATERIALS AND METHODS
A hip-resembling mould to house the authors’ ballistics gel and hip joint replica was created using a Revopoint 3D scanner (Revopoint 3D Technologies, Los Angeles, California, USA) on the torso of a general anatomy model. Using this rendering, the authors edited the torso scan in Meshmixer (Autodesk, San Francisco, California, USA) to create a fillable mould resembling the right hip. First, the authors’ used the ‘Cut’ command to reduce the print down to the necessary structures, and then the addition of superior and inferior walls created a fillable shell (Figure 1A–D).
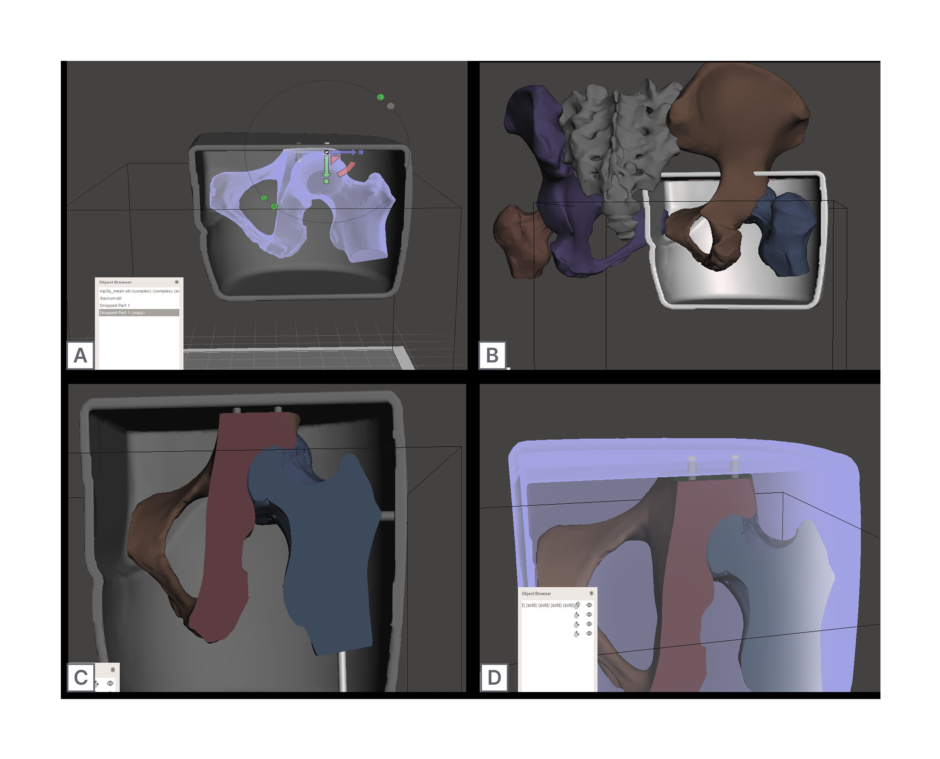
Figure 1: Computer generated designs to create a realistic 3D printed hip phantom.
A) Rendering of the entire hip joint aligned within gelatine mould cast. B) Rendering of the edited right hip joint, fitting within the edited gelatine mould cast. C) Mould cast and hip joint rendering, with associated holes generated for joint suspension. D) Final rendering of mould and hip joint renderings, with emphasising connections made by the Boolean subtracted hole function.
The hip mould was then scaled to the appropriate size using Fusion 360 (Autodesk). After scaling their hip mould rendering, the authors used a LulzBot Taz 6 3D printer (LulzBot, Fargo, North Dakota, USA) using a verbatim polylactic acid (PLA) filament (215 °C; 15% infill) to print the model. The hip mould was painted with a single layer of latex paint to ease with extraction of the final ballistics gel product.
A 3D printing template of the human hip joint was downloaded from Thingiverse9 (MakerBot Industries, New York City, USA) under the creative commons license and edited with Meshmixer software (Autodesk) to include the right hip joint and its associated structures (Figure 1A). In brief, individual components were digitally combined, scaled to the correct size, and plane cut to fit the mould. The rendering was re-meshed and, finally, Boolean subtracted holes were added to the model design to allow for bone replica suspension within the final hip model (Figure 1C and D).
A LulzBot TAZ 6 3D printer using Verbatim PLA filament (215 °C; 15% infill) was used to print the final model of the hip’s bony structures. A thin layer of 20% ballistic gel solution was applied concentrically along the femoral head and hip joint to mimic the acetabular structure and left to cool at room temperature.
The finished 3D-printed hip replica was then suspended within the watertight hip mould using toothpicks, utilising small holes created by the Boolean subtracted holes function for support (Figure 2A). A second mixture of 15% ballistic gel solution was poured into the mould and let to set overnight at 4 °C (Figure 2A). After setting overnight, the support toothpicks were extracted from the mould and the hip model was carefully removed from the latex-coated mould (Figure 2B).
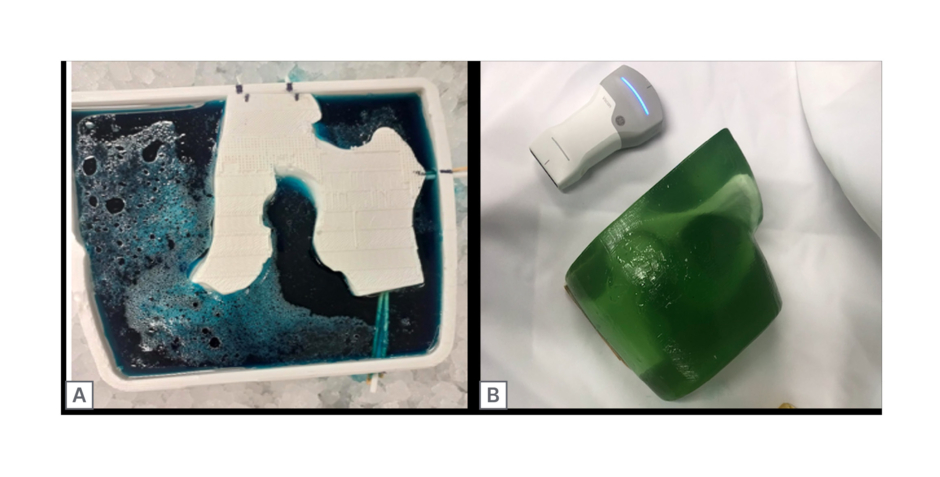
Figure 2: Poured and final prototype of ultrasound hip phantom.
A) 3D printed ballistics mould cast (blue dye), with suspended 3D printed hip replica and a 15% ballistics gelatine infill. B) Final hip phantom prototype (green dye).
The finished phantom was scanned with the curvilinear probe end of a VScan AirTM wireless US machine (GE HealthCare, Chicago, Illinois, USA), which is visible in Figures 2B and 3A.
RESULTS
The authors’ 3D printed hip phantom achieved similar anatomical and echogenic appearances to images observed of a human proximal femur, doing so at a fraction of the cost of traditional US phantoms currently on the market (Figure 3A).
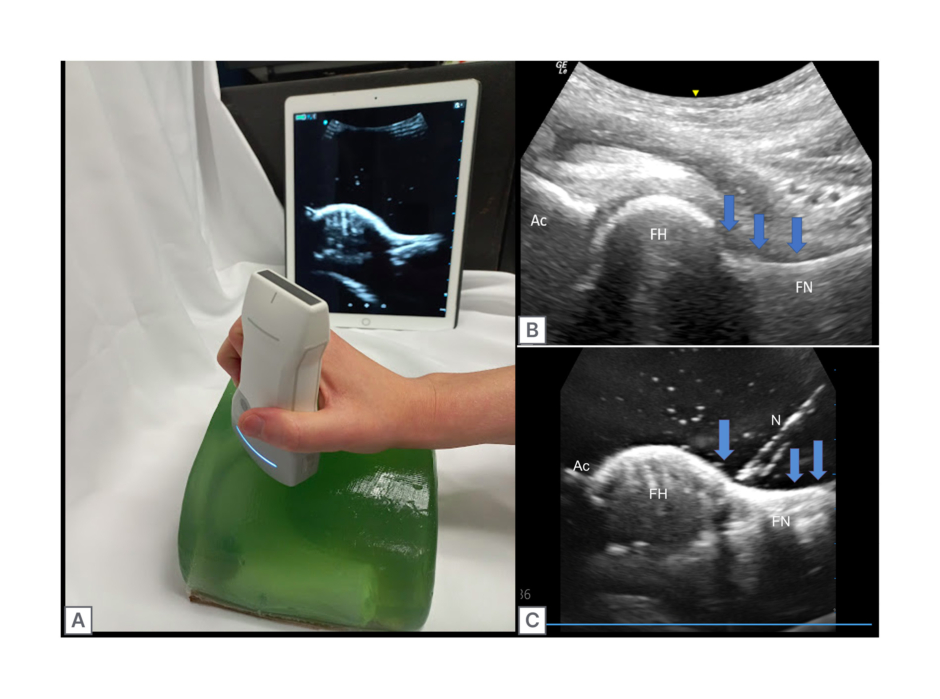
Figure 3: Pre- and post-injection ultrasound images of the 3D printed hip phantom compared with a human hip joint, with relevant features labelled.
A) Completed hip phantom with simultaneous ultrasound imaging in pre-injection state. B) Labelled ultrasound imaging of human hip. C) Ultrasound imaging of hip injection within the phantom model. The blue arrows show the joint capsule and target for the joint injection. Ac: acetabulum; FH: femoral head; FN: femoral neck; N: needle.
By utilising the art and science of 3D printing, the authors were able to achieve a good echogenic appearance of the proximal femur and its surrounding anatomical structures, making this model not only ideal due to its low cost but also as an accurate teaching tool for reviewing anatomical structures in the long axis view (Figure 3B and C). Additionally, the use of computer-aided software allowed the authors’ replication of the femoral head, neck, and its surrounding structures to be as precise and accurate as possible, while limiting the presence of back shadowing and material-induced artefacts within their scans. While artefacts from the bony replica were minimal, air bubbles were visible upon scanning of the authors’ model. These air bubbles are small artefacts caused by air trapping during the pouring of the gel into the mould and cause negligible visual obstruction.
Doubling as an injectable trainer, the authors’ hip model sufficed in its ability to display and absorb needle-injected fluids, making this phantom a valuable learning tool in simulating the visual–spatial and tactile skills needed to perform hip joint aspirations and injections within a living specimen. Repeated needle sticks and injections were possible with the authors’ phantom, leaving minimal tracking lines with each use. While this model was reusable, needle tracks deep within the gel did become obstructive to image acquisition after sustained use. These needle tracks could be removed by peeling off the gel from the 3D hip replica, heating it to a boil, and repouring the phantom using the original 3D printed mould and suspensory toothpicks.
DISCUSSION
With the growing popularity and accessibility of 3D printing technology among academic institutions and households, the utilisation of computer-generated 3D printing technology makes the authors’ low-cost US trainer easily accessible, printable, and customisable. In addition to making the authors’ model more attainable, the use of computerised 3D printing software also allows for more realistic and detailed internal lying structures within the author’s phantom, making it a more accurate training tool than others rendered from less exact methods.
An in-plane, long-axis scan was performed on both a human and the hip phantom model. This study found the hip phantom to be comparable to human models in simulating various clinical skills such as tactile awareness, visual–spatial acuity, and general sonography technique. Additionally, the hip phantom was found to be cast in a similar shape to what one would encounter during human hip sonography, further adding to its use as an accurate procedural simulator. While hip phantoms do already exist on the medical education market, these tools can cost upwards of 5,000 USD, and are often non-injectable.10,11 The authors’ model was completed with an approximate cost of 50 USD, emphasising the increased accessibility and affordability when considering using simulation models in the realm of medical education.
Due to the PLA filament’s durability in terms of being embedded in gel and exposed to needle sticks, the authors’ hip model and its casting mould can be used repeatedly, allowing for reuse and recycling of the phantom’s base for cost-efficient, hands-on educational learning, even after consistent injections and practice sessions. The ability to recast the gel within the author’s phantom also leaves room for further duality and customisation, as the user can manipulate the gel consistency and colour it to match the skill set of the trainee navigating the US. Thus, the authors’ model provides a cost-efficient, customisable format to encourage further learning in the realm of musculoskeletal sonography and skills.
LIMITATIONS
While the authors were able to create a hip phantom that is sonographically similar to images obtained from a previous human specimen scan, their phantom was not evaluated in comparison to a human model in real-time. Additionally, though the bony structures of the authors’ phantom were pulled from an accurate representation of a human specimen, some of the anatomical features in their task trainer were not translated perfectly. For instance, the sonographic appearance of muscle tissue and fat versus tendons and cartilage still provide challenges. This is also true of the 20% ballistic gel, which was used to recreate the acetabulum, as this material was not a perfect match in mimicking the structure’s qualities on a human sonographic scan, appearing less radio-opaque than its human specimen counterpart (Figure 3B and C).
Additionally, pouring of the 15% ballistic gel within the mould led to occasional air bubbles to be trapped within the phantom itself. While these bubbles did not markedly affect the sonographic imaging, these artefacts did cause a small visual impairment. Furthermore, the use of ballistic gel leads to traces or needle track marks after repeated needle sticks when simulating injections. While minimal at first, these marks increase with phantom use, thus creating increasing amounts of sonographic artefacts over the lifetime of the phantom. However, to be emphasised again, needle tracks in a 50 USD phantom are a little more forgivable than in one that costs significantly more.
While the phantom itself was injectable and held lower viscosity fluids with ease, there was no intact mechanism for emptying the area around the acetabulum or femoral head after attempted injections. This was not of issue for the authors’ number of sonographic readings but may pose a problem for use of this model in an educational, multi-simulation setting.
Lastly, the authors’ model was not tested in real-time in conjunction with a human subject. Thus, this study cannot assess or compare learner competence on a live subject subsequent to training with the phantom.
CONCLUSION
As sonography continues to grow in its use as a bedside diagnostic and procedural device, the need for US phantoms providing visual and tactile feedback for novices and training professionals becomes all the more apparent. While such simulators are currently on the market, they often come at a high cost, often deterring from their use in the realm of medical education. This manuscript outlines parameters for using 3D printing capabilities to create a versatile, low-cost, and yet reasonably accurate hip phantom while keeping accessibility at the forefront of product design. With these findings, the authors’ aim to make hands-on teaching with sonography phantoms more accessible to medical students and learners’ alike, further promoting the expanding use and expertise of this outstanding diagnostic tool in many fields to come.