Abstract
As sonography (US) continues to become a mainstay in medicine, both as a screening device and as a procedural tool, the use of task-based sonographic trainers (i.e., phantoms) in medical training has become increasingly necessary. This is especially true for what has been termed ‘the global use of US’, or the use of US as an imperative tool during structural planning and placement of peripheral and central lines within patients in emergency and hospital settings. However, despite the frequency and technicality of US-guided procedures involving arterial and venous line placement, the benefit of such hands-on US simulation training for employees and professional students alike is often outweighed by cost. The current market for US trainers displaying human forearm anatomy and branching vessels comes at a high price tag of over 2,000 USD per model. The authors’ experiment utilises 3D printing techniques and biodegradable ballistics gel to create a cost-effective, versatile, and yet realistic peripheral line simulator in order to cultivate real-time learning of arterial/venous arm anatomy, while providing mechanical practice for needle driving and US technique, all at a fraction of the commercial price.
Keypoints
1. Using ultrasound (US) guidance for procedures such as peripheral intravenous (IV) line placement, consistently yields better patient outcomes. As US-guided procedures become more clinically prevalent, the need for effective training methods has grown. US phantoms have proven to be invaluable tools for hands-on practice. Phantoms enhance learners’ confidence, proficiency, knowledge base, and skillsets for various US-guided procedures. Furthermore, these educational tools offer replicability, affordability, and reusability, making them accessible to many learners and institutions.2. This study utilised 3D printing technology and biodegradable ballistics gel to develop a realistic, reusable, and cost-effective phantom forearm for the purposes of aiding training of healthcare professionals in performing US-guided IV access.
3. Beyond US-guided IV access training, these phantoms have numerous potential applications in the medical field. These include training vascular surgeons, practising haemodialysis access procedures, simulating emergency vascular access techniques, conducting vascular research and device testing, educating patients, and facilitating radiological interventions. The adaptability of these phantoms makes them valuable tools for healthcare professionals across different specialties, enhancing medical education, research, and patient care.
INTRODUCTION
Ultrasonography (US, also known as sonographic imaging, ultrasound) is an important medical imaging technique that uses high-frequency sound waves to create images of internal body structures. It is non-invasive, painless, and does not involve ionising radiation, making it a safe and effective diagnostic tool in a wide range of medical applications. Previously described by Bercoff1 as “a disruptive technology in the medical device arena,” the realm of US within healthcare remains ever-growing, as practitioners continually innovate and repurpose the devices’ real-time anatomical imaging to provide more accurate and timely care.1 Ranging in its application from cardiographic imaging to that of obstetrics, vascular Doppler, venous access, or even emergency Focus Assessment with Sonography in Trauma (FAST) exams, US continues to show its potential as a real-time investigative and diagnostic technology capable of empowering its users with accurate, processable information, allowing for both emergent diagnosis and life-saving interventions.2
Useful in times where tissue oedema, trauma, obesity, or variances in vasculature might impede intravenous (IV) access, US has changed the way that practitioners approach complicated patient anatomy in the acute setting.3 As has been illustrated by multiple studies, the use of US in peripheral intravenous (PIV) line placements systematically provides better outcomes for patients.4 When studied in the paediatric population, it was found that US-guided IV access had an overall success rate of 80%, compared to traditional attempts with a success rate of only 64%. In the same study, US-guided line placements were found to require less overall time (6.3 minutes versus 14.4 minutes), in addition to fewer needle insertion attempts (one versus three), and fewer needle redirections while under the skin (median: two versus 10) compared to traditional techniques.5
In addition to these immediate outcomes, the use of US for line placement poses another benefit: avoidance of central line placement for access-only purposes. In a study comparing central venous catheters to PIVs in patients in the intensive care unit, the incidence of bloodstream infections within PIVs was much lower than that of central lines (0.5 versus 2.7 per 1,000 catheter days). Furthermore, while PIVs tended to acquire localised instances of infection, phlebitis, or haematoma, central venous catheters characteristically displayed more diffuse effects, putting patients at risk for more serious systemic illness. With these factors in mind, it is clear that practitioner use of US technology can aid in providing both better immediate and long-term outcomes for patients, from both a comfort and an infectious standpoint.2
As US-guided procedures become increasingly widespread in modern clinical practice, training medical professionals to be adept in these skills while using safe, standardised methods has become all the more imperative. While hospital-based learning remains a great way to facilitate these skills, quality of student training must also be considered alongside the medical facility’s primary objective, to standardise patient care and maximise outcomes.6 In a study by Passaperuma et al.,7 patients across various specialties were asked to rate their comfort levels with medical students assisting in their care. Patient comfort levels in medical student involvement were found to steadily decline with varying levels of invasiveness, with only partial comfortability (3.5 out of 5) being attributed to students’ involvement in minimally invasive procedures, such as peripheral IV placement.7 With patient beneficence in mind, the need to strive for other teaching modalities that can realistically teach the skills needed for US-guided procedures remains.
One such method of teaching sonographic skill includes the use of US trainers, or ‘phantoms’, in lieu of real human subjects. These phantoms can be made using animal tissue, elastomeric latex, or agar/guar gum based gelatines.8,9,10 In order to properly mimic human anatomical structures, a US phantom should maintain certain characteristics, such as displaying similar echogenicity to human tissues, being readily available, being inexpensive, and proving tactile feedback to users.11 Additionally, a phantom trainer should ideally be reusable, avoiding the generation of needle track marks after use. Through the use of US phantoms, learners can receive hands-on, interactive practice without any danger and discomfort for a living patient.10 Previous studies have shown that the use of US phantoms significantly improves not only the confidence and proficiency of novice learners, but also their underlying knowledge base. 12,13 Additional studies have also found quantifiable improvements in techniques involved in nerve blocks,14 lumbar facet joint injections,15 and general needle-dexterity16 in students who have been trained on US phantoms. These findings of increased competency and skill have further added to the emphasis on US education within the medical school curriculum, as countless studies continue to mark the importance of such teaching modalities within the preclinical setting.17,18
Given the growing popularity of US within critical and primary care, many companies have begun manufacturing US phantoms for various skill-training purposes, including practising hip joint injections, central venous access, and nerve blocks. However, their use in the medical education setting is often outweighed by cost, as phantom models can range in price to upwards of 1,000–4,000 USD.19,20 In addition to cost, (commercial) phantoms are also limited in their use due to non-modifiable characteristics, such as phantom opacity to match the knowledgebase of the learner, or materials prone to tracking marks following repeated needle sticks.
This paper aims to develop a realistic, affordable, and reusable arm phantom for training medical providers in the technique of US-guided forearm IV access. Currently, existing versions of such devices are cost-prohibitive to many learners and institutions, and often do not display accurate, distinguishable arterial and venous anatomy within. The authors propose a new, realistic model of the human forearm using 3D printing alongside ballistics gelatine to ensure standardised, affordable learning for healthcare professionals, all at a fraction of the commercial price.
MATERIALS AND METHODS
A rendering of a human forearm and elbow was created by imaging a human arm with a Revopoint POP 3D Scanner (Revopoint3D Technologies Inc., Shenzhen, China). This rendering was then edited in Meshmixer (Autodesk, San Francisco, California, USA), using Boolean difference, plane cut, and remeshing functions to create a hollow forearm mould for superficial structures (Figure 1A). Next, a Lulzbot Taz 6 3D printer (LulzBot, Fargo, North Dakota, USA) using Verbatim polylactic acid filament (Verbatim, Charlotte, North Carolina, USA; 215ºC, 15% infill) was used to print the forearm mould, which was then affixed and painted with latex to account for printing artifacts. A thin coat of vegetable oil was then applied to ease the eventual extraction of gelatine from the mould.
Following the creation of the arm mould, vascular structures were assembled using two different types of latex tubing, which were selected to highlight differences in size, thickness, and compressibility of arteries versus veins. The authors selected slightly more rigid and thicker walled tubing for arteries, and thinner walled, very flexible tubing for veins. With these materials, two separate branching vessels resembling human forearm vasculature (arterial versus venous) were made by cutting and affixing the tube ends at 30-degree angles with surgical scissors and DERMABOND® glue (Ethicon, Bridgewater, New Jersey, USA) (Figure 1B). These vessels were subsequently filled with red and blue dyed water, capped using latex plugs to mimic arterial versus venous fluid, and suspended within the arm mould using toothpicks (Figure 1C).
To create the gelatine, 400 mL of water was heated on a hotplate, slowly mixed with 100 g ballistic gelatine powder until the gelatine was dissolved, and volume adjusted to 500 mL total, resulting in a 20% mixture (weight/volume). The ballistics gelatine mixture was subsequently poured into the forearm mould, with the branching vessels suspended in the gel using toothpicks. The mould was allowed to set overnight in a refrigerator. The resulting phantom was removed from the mould using a basic butter knife (Figure 1D).
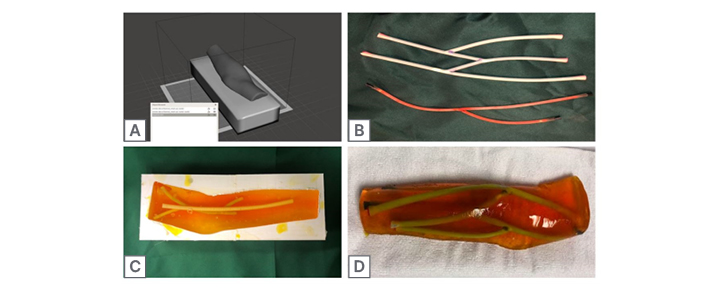
Figure 1: Trainer creation.
RESULTS
Utilising 3D printing and ballistics gelatine, the authors’ arm trainer was able to achieve good echogenic appearance of various vessels, in addition to housing these vessels within a contour anatomically similar to that of a human forearm. Using a GE Venue 40 US machine (GE HealthCare, Chicago, Illinois, USA) with 12L linear probe, the model’s embedded vessels were appreciated in both long and short axis views, allowing for visualisation of branching vessel structures and needle stick simulations (Figure 2A–D). These views were found to be relatively clear, and with minimal artifacts such as bubbles in the gelatine. There was also an appreciable variance in vessel wall thickness and compressibility, mimicking the ‘floppier’, more compressible nature of human veins (Figure 2C and 2D) in direct comparison to their thicker arterial counterparts (Figure 2A and 2B).
Using US, the authors were able to track the model’s vessels along various branching points throughout the forearm, allowing for the user to both review vascular anatomy and practise needle sticks simultaneously. The authors’ model also allowed for visualisation and practice of injecting branching vessels at various depths.
Simulation of fluid injections and aspirations was possible with this model, allowing for immediate tactile feedback resembling that of entering human tissue and vasculature. Specifically, the model allowed for practice in identifying needle sticks within the long axis view, as well as simulating fluid flow during an injection into the vascular structure (Figure 2E–G). In addition to allowing for the mechanical practice of fluid injection, the authors’ branching vessels also displayed red and blue fluid solutions when aspirated to further characterise arterial versus venous structures to the learner (Figure 3A and 3B).
Finally, the authors’ model (Figure 3C–F) was studied in direct comparison to the vasculature of a human specimen, showing similar contour of branching veins within the greater anatomy of the human forearm (Figure 3G–J).
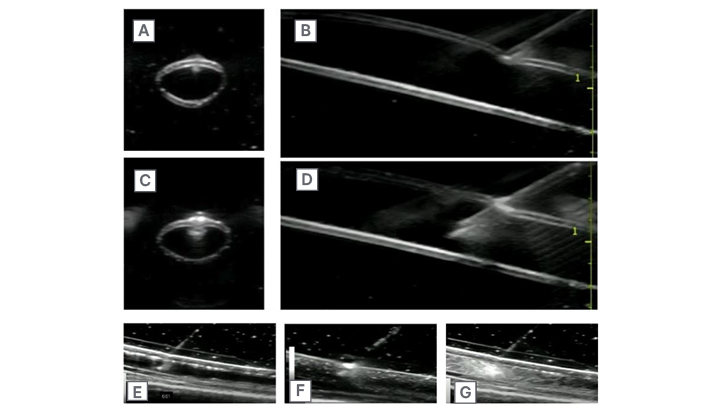
Figure 2: Simulated vessels and injection.
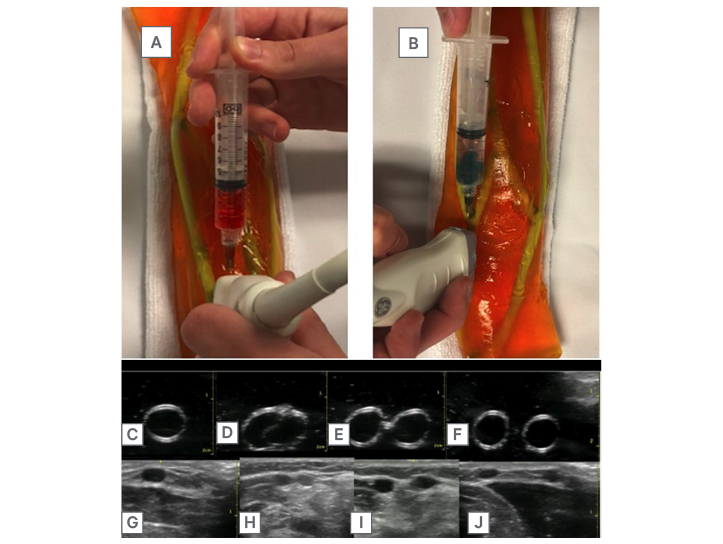
Figure 3: Fluid aspiration and comparison of trainer with human arm.
DISCUSSION
With 3D printing, latex tubing, and ballistics gelatine, the authors have successfully created a working phantom prototype of a human forearm that can be replicated and altered based on user needs. This model showcases an overall structure mimicking that of a human forearm, and its gelatine can be dyed with varying levels of opacity to match the skill level of its learner. In addition to anatomically sound outer dwelling characteristics (antecubital fossa, lateral and medial epicondyles), the authors’ model also houses multi-branching vascular structures designed to further simulate the true human vasculature of the forearm, allowing for anatomical practice for learners with every use. Concordantly, the branching vessels of the trainer allow for further learning in sonographic tracking along human vasculature, allowing for practice in the localisation of ideal entrance points for IV access along branching and otherwise complicated circulation. Finally, the authors’ decision to use slightly thicker latex tubing for arteries and thinner tubing for veins enabled them to replicate variations in vessel compression realistically. This approach effectively emulated the greater compressibility of veins compared to arteries, a distinguishing feature commonly utilised to differentiate these vascular structures.
In addition to its anatomical likeliness to that of a human subject, the vasculature residing within the model is suspended at various depths in order to allow for practice of superficial versus deep sonographic skills and needle sticks. With this feature, the user may practise how to navigate around important overlying structures when accessing imperative vessels underneath. These branching vessels also possess varying qualities in thickness and compressibility, allowing for simulation of compression skills via US to distinguish between arterial (non-compressible) and venous (compressible) structures. Beyond compressibility, this model allows for the user to check their skills with immediate colour feedback, as arterial structures aspirate with red fluid, and venous with blue. These aspirations can be viewed by the user in short axis and long axis views, allowing learners to actively practise tactile feedback and needle driving techniques alongside that of US.
Beyond the immediate learning benefits of the arm model, the authors’ project highlights another benefit to learners: replicability and affordability. Rendered and created with 3D printing technology, the base to this arm trainer is easily re-printable at any facility with a 3D printer and filament, allowing for widespread use of the model within the educational setting. Additionally, having been printed in polylactic acid filament, the printed arm mould is strong and temperature resistant, allowing for multiple castings of new models with repeated effect. The model is also made of a standard ballistics gelatine, which can be bought in bulk, or remelted and repoured into the original mould to renew original, artifact-free phantom architecture. With a base material cost of approximately 50 USD, this model competes with others in the commercial sector, both in initial cost and in reusability.
LIMITATIONS
While the authors were able to create a US trainer of the forearm specifically meant for the practice of US-guided injections, the needle-accepting qualities of the phantom were not evaluated in comparison to that of a human model in real-time. Additionally, though the vasculature simulated within the model was similar in size and compressibility to that of the human arm, the depth of these simulated veins varied significantly from that of a human subject (2.5 compared to 1.8 cm in human subjects).
In conjunction with these subtle variances in vascular depth, the model also sustained some anatomical features that did not translate perfectly into the final edit. While 20% gelatine was used to create the bulk of the model, this material showed occasional signs of artifact through air bubbles. The gelatine used was also unable to perfectly mimic the intricate musculature of the human arm as seen in US. Additionally, the gelatine was found to have needle artifacts following continued use, thus necessitating replacement of the gelatine following many uses.
In practical use, the model was able to both accept and aspirate fluids from veins accordingly. However, the current model does not possess a method for refilling the vasculature with coloured fluid, nor does it have a way to remove liquids that might have been injected outside of the vascular branches. Though minimal, fluid buildup could lead to further artifacts seen on repeated US scans. With continued use of latex tubing for the vascular structures, it was found that the latex was not always watertight, necessitating switching out of the branching vessels after multiple uses due to leakiness from perforations. This is a common problem with blood vessel trainers, and the authors have not been successful in solving this issue yet. However, they have recently identified a company that sells a ‘self-healing’ phantom for 468 USD (VATA, Canby, Oregon), which, unfortunately, is once again cost-prohibitive for many users. It appears that the ‘self-healing’ ability would require a change in the materials used in the manufacturing process, driving the cost up. Even if the phantoms had self-healing vessels, the authors would still deal with needle tracks after repeated sticks, which would minimise the benefit of self-healing vessels.
Finally, the arterial and venous structures were not pressurised within the model, and were therefore not able to simulate the fluid ‘flash’ associated with perforating a human vessel with a needle.
CONCLUSION
The goal of this project was to develop a realistic, reproducible, reusable, and affordable phantom arm model for training healthcare professionals in US-guided PIVs. Using 3D printing, ballistics gelatine, and readily-available materials, the authors’ team successfully produced a prototype with characteristics that will facilitate training in the PIV insertion procedure. These characteristics include a realistically-shaped, echogenic medium; branching, fluid-filled vessels in anatomic location; ability to aspirate fluid from the vessels with colour feedback denoting artery versus vein; compressible tubing which highlights arterial versus venous response to applied pressure; and relative affordability compared with existing, similar products. Importantly, the authors believe there are many additional potential applications beyond peripheral IV access to explore that the phantom might be adapted for (naturally this will require further experimentation). The authors believe this not only highlights the versatility of the model, but also sparks further interest in its utility. Some potential applications include vascular surgery training, as aspiring vascular surgeons could benefit from using the phantom for training purposes. It could possibly be adapted to replicate different vascular pathologies and surgical scenarios, providing a valuable platform for skill development. For haemodialysis access training, the creation of arteriovenous fistulas and grafts for haemodialysis is a critical procedure. The phantom could potentially be customised to simulate these access points, enabling healthcare professionals to practise and refine their skills. It could also be used for emergency procedures: in emergency medicine, healthcare providers often need to rapidly establish vascular access in critical situations. The phantom can serve as a tool for training in emergency vascular access techniques, such as intraosseous needle insertion.
Beyond education and training, the phantom can be used for research purposes, such as vascular research and device testing. It provides a consistent and controlled environment for testing vascular devices, such as catheters, stents, or embolisation materials, before their clinical application. The phantom can also be employed as a patient education tool. It can help healthcare providers explain vascular procedures to patients and their families, enhancing their understanding and reducing anxiety. Finally, radiologists performing vascular interventions, such as angiography or embolisation, can use the phantom to simulate complex vascular structures and refine their skills in a safe environment.
In conclusion, the versatility of the phantom extends beyond peripheral IV access, encompassing a wide range of applications in medical education, training, research, and patient care. Its adaptability makes it a valuable tool for healthcare professionals across various specialties. Although minor limitations remain, this prototype is a breakthrough in the widespread effort to increase the quality and efficiency of modern medical education.