Meeting Summary
Several presentations at the recent International Liver Congress™ (ILC), held in London, UK, from 22nd–26th of June 2022, addressed the role of the gut microbiome in chronic liver disease. Debbie L. Shawcross from the Department of Inflammation Biology, School of Immunology and Microbial Sciences, Institute of Liver Studies, King’s College London, UK, outlined the role of the gut-liver axis in the pathogenesis of cirrhosis, and how existing and novel therapies manipulate gut microbes.
Emina Halilbasic from the Medical University of Vienna, Austria, and Benjamin H. Mullish from the Division of Digestive Diseases, Imperial College London, UK. Focused on the use of gut-based therapies in cholestatic liver disease. They explained the current understanding of the interplay between bile acids, microbiota, and the mucosal immune system, and the ways in which this may be manipulated for therapeutic gain.
The role of gut barrier impairment in alcohol-related liver disease (ArLD) was presented by Shilpa Chokshi from the Roger Williams Institute of Hepatology, Foundation for Liver Research, London, UK, and School of Immunology and Microbial Sciences, Faculty of Life Sciences and Medicine, King’s College London, UK. Charlotte Skinner from the Department of Metabolism, Digestion, and Reproduction, Division of Digestive Diseases, Imperial College London, UK, described the role of gut proteases in this process, while Jasmohan S. Bajaj from the Virginia Commonwealth University, Richmond, USA, and Central Virginia Veterans Healthcare System, Richmond, USA, illustrated new therapies that target the gut-liver axis in this condition.
Yue Shen from Zhongshan Hospital, Fudan University, Shanghai, China, and the Department of Gastroenterology and Hepatology, Shanghai Institute of Liver Diseases, China, described a combined microbiome-metabolome study to characterise the gut microbiome in hepatitis B virus infection-associated liver diseases (HBV-CLD), and how specific microbes might impact peripheral immunity. Finally, Bajaj outlined why the gut is a major target for hepatic encephalopathy (HE) treatment and described cutting edge research into therapies that show promise in this arena, such as soluble solid dispersion rifaximin, faecal microbiota transplantation (FMT), and rationally defined bacterial consortia. Overall, these presentations highlight an expanding knowledge of the gut-liver axis and promise an exciting future in liver treatment.
Role of the Gut–Liver Axis in Cirrhosis
Debbie L. Shawcross
The gut and liver are intimately linked, since blood that infuses the liver has first passed through the gut (Shawcross DL et al., ILC 2022, unpublished data). Shawcross emphasised an increasing interest over recent years in the gut microbiome and its multifaceted impact on the body, leading to the moniker ‘the second genome’.1 Biochemical signals from gut microbes impact metabolic pathways in the human body, with diet, genetics, and birth route all impacting the diversity of their microbiome.2
A number of mechanisms link the microbiome to the development of hepatic steatosis, including appetite regulation, energy extraction from the diet, endotoxin release, insulin resistance, ethanol production, short-chain fatty acid (SCFA) synthesis, and bile acid homeostasis ( Figure 1).3
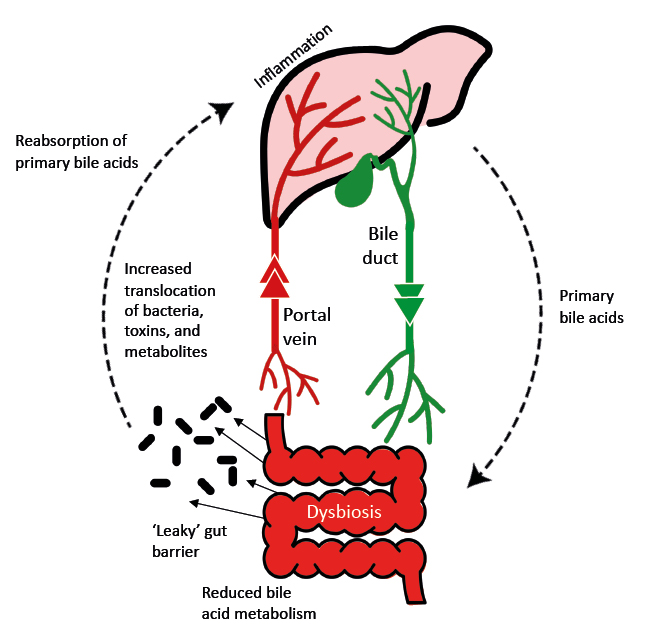
Figure 1: The gut-liver axis in liver disease.
Adapted from Blesl et al.4
Some types of gut bacteria can induce liver injury, and Shawcross presented the example of a study of faecal samples from patients with alcoholic steatohepatitis, where patients had 2,700-fold more Enterococcus faecalis compared with samples from controls.5E. faecalis can produce the exotoxin cytolysin, which has lytic activity against both Gram-positive bacteria and eukaryotic cells. Within 180 days after hospital admission, cytolysin-positive faecal samples were associated with a significantly higher mortality rate compared with cytolysin-negative samples (89.0% versus 3.8%, respectively; p<0.0001). In a germ-free mouse model, E. faecalis colonisation was found to compound the increase in hepatic triglycerides and serum alanine aminotransferase that followed alcohol-induced liver injury.5
Gut dysbiosis, including the loss of symbiotic microbes, an increase in pathogenic microbes, and a loss of microbiome diversity, has been considered a causative agent of cirrhosis.6 One of the first studies to make this connection was published by Bajaj et al.,7 who showed that dysbiosis correlates with plasma endotoxin levels and 30-day mortality and was greater in patients with cirrhosis who develop complications.
Cirrhosis has also been associated with a ‘leaky’ gut barrier, which permits the passage of bacteria into the portal vein and thence to the liver, inducing inflammation.4 In addition to the presence of microbes themselves, serum levels of microbial metabolites have also been independently associated with acute-on-chronic liver failure and mortality in patients with cirrhosis.8
Shawcross introduced the MICROB-PREDICT study, which aims to identify microbiome-associated biomarkers associated with end-stage liver disease. This knowledge is intended to improve stratification of patients with cirrhosis, enabling personalised microbiome-based treatment. MICROB-PREDICT plans to validate several novel microbiome-based biomarkers in a clinical study and to translate these into new clinical tests for patients.9
Shawcross outlined some of the current and future approaches to manipulate the gut microbiome in cirrhosis, including antibiotics such as rifaximin, and FMT (Shawcross DL et al., ILC 2022, unpublished data).
In a single-centre, double-blind, randomised trial in patients with cirrhosis and chronic hepatic encephalopathy (n=38), rifaximin reduced gut-derived systematic inflammation and infection.10 Patients treated with rifaximin for 90 days had a reduced risk of infection (odds ratio: 0.21; 95% confidence interval: 0.05–0.96), reduced circulating neutrophil toll-like receptor-4 expression, and reduced serum TNF-α levels compared with those receiving placebo.11 Examination of the oral and gut microbiome identified orally originating bacteria in cirrhotic faeces, including Streptococcus and Veillonella species.10 Shawcross explained that these species of bacteria produce sialidases, which enable them to degrade mucin in the gut wall. Rifaximin was able to reduce these harmful populations, which could potentially reduce injury to the gut epithelia.10
Several studies have been conducted over the last few years investigating the safety of FMTs in patients with liver disease.11-13 The PROFIT study looked at the impact of FMT to the small bowel in patients with cirrhosis.14 Shawcross explained that early results suggest that FMT significantly increased microbial diversity in the gut within 7 days compared with placebo, with variable decline in engraftment between patients over the 90-day follow-up period (Woodhouse, unpublished data). Data also indicate reduction in blood neutrophils, indicative of decreased inflammation; reduction in intestinal fatty acid binding protein 2 in the stool, indicative of decreased intestinal epithelial shedding; and reduction in the pathogenic bacteria E. faecalis and enteropathic Escherichia coli. (Woodhouse et al., unpublished). Based on patient feedback, an oral capsule is preferred to an enema, a large, 5-year trial (the PROMISE study) is currently being planned in the UK, which aims to investigate the efficacy of FMT capsules in patients with cirrhosis.15
CHOLESTATIC LIVER DISEASE
Therapeutic Advances in Cholestatic Liver Disease: Bile Acids to Bowel and Back Again
Emina Halilbasic
In addition to their role in the absorption or elimination of dietary fat in the intestine, bile acids are involved in inflammation and mucosal homeostasis, and are metabolised by gut bacteria (Halilbasic E et al., ILC 2022, unpublished data).16 The regulation of de-novo bile acid synthesis involves dedicated bile acid receptors such as farnesoid X receptor (FXR) and G-protein-coupled bile acid receptor (TGR5). Other, non-specific bile acid ‘sensors’ such as pregnane X receptor, vitamin D receptor, and constitutive androstane receptor are involved in promoting bile-acid detoxification to protect tissues.16
The pathophysiology of chronic cholestatic liver diseases primary sclerosing cholangitis (PSC) and primary biliary cholangitis (PBC) involves the immune-mediated injury of bile ducts resulting in a decrease in bile flow (cholestasis).17 The resultant accumulation of bile acid in the liver leads to chronic inflammation, necrosis, and organ failure.16
Halilbasic explained that bile acid modification represents one of the major treatment targets in cholestatic liver disease, alongside the reduction of inflammation, necrosis, and fibrosis, and the restoration of the intestinal barrier.16 Bile acid-related treatments aim to reduce hepatic bile accumulation, bile acid toxicity, and inflammation whilst promoting bile flow.18 Potential targets include FXR, fibroblast growth factor 19 (FGF19), sodium-dependent taurocholate co-transporting peptide, and apical sodium-dependent bile acid transporter (ASBT, also known as IBAT).18
Ursodeoxycholic acid (UDCA) has been used for many years to treat cholestatic disorders and is used as first-line therapy in PBC.16,19 It stimulates hepatocellular and cholangiocellular secretion of bile acids and has antiapoptotic effects.19-21 Some studies have indicated that UDCA may have a beneficial effect on liver function in patients with PSC; however, it has been associated with severe adverse effects and is no longer recommended as a treatment for this disease.22,23
There is clearly an unmet need for effective therapy in PSC, and one promising drug being assessed is 24-norursodeoxycholic acid (norUDCA).24 A homologue of UDCA, norUDCA leads to flushing of bile ducts through a process of cholehepatic shunting and stimulation of bicarbonate secretion.24 A Phase II study patients with PSC indicated that norUDCA significantly reduced alkaline phosphatase (ALP) levels in a dose-dependent manner, and was well tolerated.25 A Phase III trial is currently ongoing (NCT03872921).26
Halilbasic explained that therapies such as FXR agonists, FGF19 analogues, and ASBT inhibitors all counteract cholestasis by reducing the bile acid pool.26 FXR is mainly expressed in the liver, kidney, intestine, and adrenal gland, with lower levels of expression in adipose and cardiac tissue.27 The best known FXR agonist is obeticholic acid, an approved second-line therapy in PBC that has also been shown to be effective in PSC.28 The most common adverse effect of obeticholic acid is pruritis, which occurs in up to two-thirds of patients.28,29 Another FXR agonist, cilofexor, has also been shown to be effective and generally well tolerated in a Phase II study in patients with PSC.30 However, Halilbasic pointed out that although the bile acid pool was significantly reduced with cilofexor in this study, changes in bile acid composition were minimal. Like obeticholic acid, cilofexor is currently being assessed in patients with PSC in a Phase III study (NCT03890120).31
FGF19 is an endocrine hormone produced in the intestine that, in response to FXR activation, acts in the liver to suppress bile acid synthesis.32,33 NGM282, an engineered analogue of FGF19, was effective in reducing ALP, transaminase, and immunoglobulin levels, and was well tolerated in a Phase II study in PBC.33 In PSC, NGM282 decreased bile acid synthesis and improved fibrosis biomarkers but did not affect ALP levels.34
Inhibitors of ASBT interrupt enterohepatic circulation of bile by reducing serum bile acids and increasing faecal bile acid loss, thus reducing the overall bile acid pool.35 Halilbasic explained that there are two ASBT inhibitors currently used to treat pruritis in cholestatic disease: odevixibat, approved for use in progressive familial intrahepatic cholestasis;36 and maralixibat, approved for use in cholestatic pruritis (in the USA only).37 ASBT inhibitors are promising drugs for both PBC and PSC, and clinical trials are being conducted in patients with these conditions (NCT02061540, NCT01904058, NCT02966834, NCT05050136, and NCT04663308).38-42
Finally, Halilbasic described TGR5 as another potential therapeutic target in cholestatic disease. TGR-5 is a membrane-bound bile acid receptor expressed in the gallbladder, where it modulates bile acid production; the liver, where it suppresses inflammation; and the gut, where it regulates metabolism and inflammation.43
In summary, Halilbasic reiterated that therapeutic targeting of bile acid signalling forms part of standard of care in cholestatic disease. Large Phase III studies of norUDCA and cilofexor are ongoing and offer hope for PSC treatment, but the treatment of complex cholestatic diseases may require combination therapy to achieve maximum benefits. Halilbasic posited that combinations of cholehepatic and/or antifibrotic drugs could result in synergistic or additive effects in chronic cholestatic liver disease.
Can Microbial Therapies Be Repurposed from Inflammatory Bowel Disease to Cholestatic Liver Disease?
Benjamin H. Mullish
The gut microbiome has a complex interaction with the host immune system, and gut microbial metabolites are a key intermediary in the gut-liver axis (Mullish BH et al., ILC 2022, unpublished data).16,44 These metabolites can be microbiota-derived, such as SCFAs, or host-derived and modified by the gut microbiota such as bile acids.16 In terms of cholestatic liver disease, a three-way model has been proposed to describe the interplay of bile acids, microbiota, and the mucosal immune system (Figure 2).16
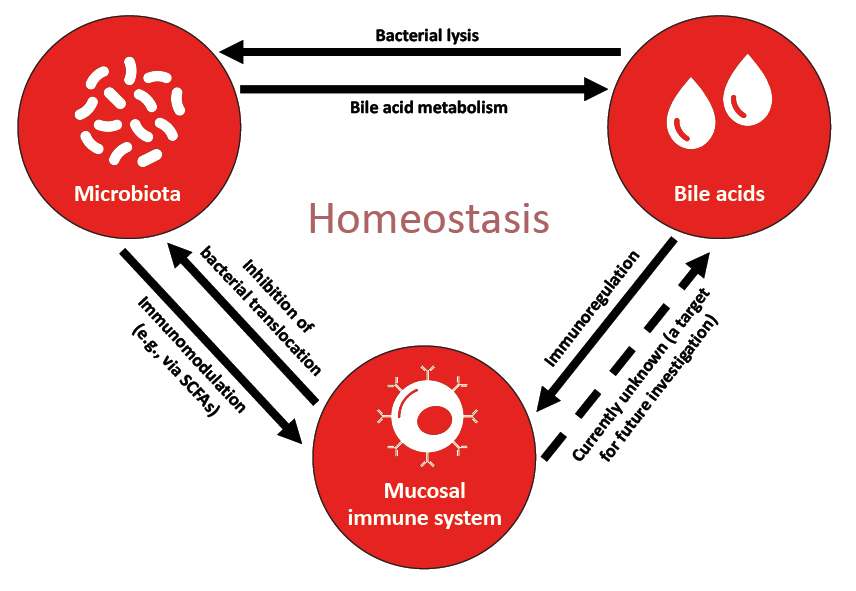
Figure 2: A model describing the interplay between bile acids, microbiota, and the mucosal immune system.
Adapted from Chen et al.16
SCFA: short-chain fatty acid.
PSC is associated with a distinct gut microbiome ‘signature’, whether or not a patient also has inflammatory bowel disease (IBD).45,46 Studies have shown that PBC can be associated with an overgrowth of Enterococcus, Fusobacterium, Lactobacillus,45Veillonella,46,47 and Klebsiella,48 and increased levels of microbial enzymes associated with bile acid metabolism.49 However, the specific taxonomic signature of the condition appears to differ between patient cohorts.45,46
Mullish described three therapeutic approaches to microbiome modulation in PSC: probiotics, antibiotics, and FMT.
Treatment with broad-spectrum antibiotics appears to worsen disease in animal models of PSC, increasing hepatic bile acid concentration, bile duct barrier dysfunction, and fatal liver injury, at least partly attributable to reduced ileal FXR signalling.50 In antibiotic-pre-treated PSC mouse models, hepatic translocation with E. faecalis and E. coli has been observed, as well as in controls.51 One potential extrapolation from this animal study is that clinicians should exercise caution in administering broad-spectrum antibiotics to patients with PSC due to the risk of exacerbating the disease. In the same experiment, concurrent colonisation with SCFA-producing Lachnospiraceae inhibited the translocation of E. faecalis and E. coli, indicating that restoration of a pre-morbid gut microbiome may have potential as an effective strategy for PSC therapy.51
In regard to establish clinical data, a case series of nine patients with ulcerative colitis-associated PSC found that vancomycin treatment for 8 weeks induced remission of colitis,52 and a systemic review and meta-analysis of the effect of antibiotic treatment (metronidazole, rifaximin, or vancomycin) on ALP in patients with PSC showed that most studies were associated with a reduction in ALP.53 Mullish described an ongoing trial being conducted in Birmingham, UK, investigating the mechanistic effects of vancomycin treatment on the gut microbiome, microbiome-mediated bile acid metabolism, and colon transcriptome in patients with IBD‑associated PSC (NCT05376228).54
Mullish explained that despite the beneficial effects of probiotics reported in IBD (particularly related to pouchitis), studies into potential benefits in PSC have not been promising. For example, a pilot study in 14 patients with IBD-associated PSC failed to show positive effects on symptoms, liver function, or liver biochemistry.55
FMT appears to induce remission of colitis in patients with UC (at least comparably to pharmacological therapy),56 and promising results were shown using capsulised FMT in this population.57 However, Mullish stressed that there is limited data for FMT in PSC so far. A pilot study of FMT from one healthy donor into 10 patients with IBD-associated PSC suggested that this may be a well-tolerated, effective treatment, with no adverse safety signals and a ≥50% reduction in ALP in three out of 10 patients and increased microbial diversity in all patients.58 A Phase II trial in PSC, the FARGO trial, will soon begin recruitment in the UK, with the aim of assessing the potential of FMT to impact the progression of the disease, and improve patients’ quality of life. Secondary outcomes will include the evaluation of the impact of FMT upon interactions between the gut microbiome, bile acids, and the immune system.
ALCOHOL-RELATED LIVER DISEASE
Gut Barrier Impairment as Key Disease Driver in Alcohol-Related Liver Disease
Shilpa Chokshi
Chokshi described the gut barrier as a multifactorial system, consisting of integrated physical, biological, and immunological components such as goblet cells, enterocytes, stem cells, paneth cells, microfold cells, dendritic cells, and innate and adaptive immune cells (Chokshi S et al., ILC 2022, unpublished data).59
Several studies have contributed to the current understanding that gut barrier failure is causatively linked to the progression of ArLD. For example, associations have been demonstrated between alcohol consumption and gut microbial dysbiosis, liver injury, intestinal cell apoptosis, and degradation of tight junction proteins.60 Moreover, gut barrier impairment may be a driver for organ damage in some people with alcohol-use disorder.61,62
In addition to the direct effects of alcohol on enterocytes,63 alcohol contributes to microbiome dysbiosis, with an overgrowth of pathogenic species, as well as having direct effects on intestinal stem cells.59,64 Acetaldehyde, an ethanol metabolite, has been shown to disrupt tight junction proteins and induces epithelial inflammation and oxidative stress.65,66 Chokshi explained that, together, these effects lead to a loss of intestinal immunity, resulting in the pathological translocation of bacterial products and proinflammatory cytokines into the lymphatic and vascular system.
Chokshi described the therapeutic goals for ArLD in the gut are to restore ‘healthy’ microbiomes, repair gut integrity, reinvigorate anti-bacterial mucosal immunity, and/or dampen injurious gut inflammation. The main approaches to improving gut barrier function in ArLD are abstinence from alcohol, FMT, and probiotics; however, Chokshi described some novel therapeutic targets identified over the past few years:
Mucosa-associated invariant T cells are involved in the homeostatic control of gut flora and in antibacterial mucosal defence. They are found in the lamina propria of the gut, but also in the liver and peripheral blood. There is a significant depletion of mucosa-associated invariant T cells in the gut and systemic circulation of patients with ArLD, particularly in those with alcoholic hepatitis.67
Immunoinhibitory receptors programmed death 1 and T cell immunoglobulin and mucin-domain-containing molecule are associated with increased bacterial translocation in patients with ArLD.68 Inhibition of these receptors may be able to restore antibacterial defences.69
Metformin has been shown to modify the gut microbiome and improve leaky gut syndrome in patients with Type 2 diabetes.70
Chokshi concluded that the gut appears to be the first site in the body to be damaged by alcohol in the development of liver disease, but that more work needs to be done to develop effective therapies targeting gut barrier function to reduce ArLD progression.
Tight Junction Damage and Increased Gut Permeability in Alcohol-Related liver Disease May Be Mediated by Gut Proteases
Charlotte Skinner
Skinner emphasised that the mechanisms by which bacteria and bacterial products translocate from the gut to the portal vein in ArLD are not fully understood. Apart from potential direct effects of alcohol and virulence factors from gut microbes, she explained that microbiota-associated proteases may play a role in this mechanism.71
Skinner described an ex vivo study that aimed to explore this possibility. In this study, faecal water was extracted from stool samples from patients with ArLD (n=25) and healthy controls (n=13), and protease activity in the faecal water was assessed using fluorescently labelled casein. Madin–Darby canine kidney cells (MDCK) a mammalian endothelial cell line) and Caco-2 cells (a human colorectal adenocarcinoma cell line) were grown to confluent monolayers on trans-well inserts to model the gut barrier. Faecal water samples were applied to the apical surface of the insert, with or without a protease inhibitor. After 120 minutes, transepithelial epithelial resistance (TEER) was used to estimate cellular permeability. Results showed that increased protease activity in faecal water correlated with a reduction in TEER for all subjects (r=-0.5888; p<0.001), and that a protease inhibitor abrogated this correlation. Change in TEER also correlated with the liver function of the individual from which the faecal water originated, as measured by the Model for End-Stage Liver Disease (MELD) score (r=-0.472; p=0.0036).71
Faecal water samples were serially diluted and plated on lactose-free semi-skimmed milk to isolate protease-producing bacteria. DNA sequencing identified resultant colonies as E. faecium, Shigella flexneri, and Staphylococcus epidermidis. The supernatant from these colonies was able to induce similar changes in TEER to faecal water samples.71
To explore the effect of proteases on tight-junction proteins, faecal water was applied to MDCK monolayers, which were then stained with antibodies against zonulin-1. Staining was markedly decreased in MDCK cells treated with high protease-containing faecal water from patients with severe alcohol-related hepatitis compared with low-protease controls (p=0.0097). Skinner explained that this suggests faecal water proteases have a destructive effect on tight-junction proteins. This finding was corroborated by the staining of mucosal duodenal samples from patients with ArLD (n=5) with zonulin-1 and claudin-1 proteins, which was weaker in patients with decompensated versus compensated cirrhosis.71
Skinner concluded that protease activity in patient faecal water can cause tight-junction damage and increased gut permeability in vitro, which correlates with liver function. This suggests a potential mechanism by which gut-derived virulence factors reach the liver in patients with ArLD.
New Treatments that Target the Gut-Liver Axis in Alcohol-Related Liver Disease
Jasmohan S. Bajaj
Bajaj emphasised that apart from the impact on intestinal permeability, alcohol misuse is associated with brain dysfunction, and in those patients who go on to develop ArLD, there is also evidence of an altered circadian rhythm (Bajaj JS et al., ILC 2022, unpublished data).72 Bajaj emphasised that these issues lead to a vicious cycle of worsening brain dysfunction, alcohol abuse, steatosis, and fibrosis. Microbiota are associated with many aspects of this cycle, indicating that alterations in the structure and function of the gut microbiota are critical to understanding the risk of developing ArLD and disease progression.72
Many of the current drugs that support alcohol abstinence are suitable for use in patients with cirrhosis.73 However, because they are commonly associated with adverse effects, Bajaj stressed that many clinicians try not to prescribe them. For example, studies have shown that treatment for alcohol-use disorders reduces the development of hepatic decompensation, 180-day mortality rate, and long-term mortality rate;74,75 however, only 14% of patients received any treatment, and just 1.4% received medical-associated therapy.74 When the reasons for these limited treatment figures was analysed, it was found that 107 out of 264 patients refused treatment when it was offered to them.76 Bajaj emphasised the importance of finding other ways to engage these patients, and gut-liver axis therapies may offer a potential solution.
Gut-liver axis therapies focus on reducing alcohol cravings or harmful intake, improving liver-related outcomes, and improving alcohol-related outcomes. A Phase I study of FMT in patients with alcohol-use disorder and cirrhosis found that, after taking FMT for 15 days, alcohol cravings and consumption, systemic inflammation, and intestinal permeability were decreased, and quality of life and cognition were increased, compared with placebo.77 These changes were associated with an increase in microbial diversity, SCFA-producing microbes, and plasma and stool SCFAs, Bajaj highlighted that the long-term 6-month follow-up data was particularly striking, showing a lower incidence of alcohol-use disorder-related hospitalisations and severe adverse events in patients that received FMT compared with those who received placebo. Human-to-mouse experiments indicated that the microbiome of patients with alcohol-use disorder and cirrhosis had a substantial effect on the gut transcriptome in mice, compared with the microbiome of these same patients after receiving an FMT from a healthy donor.78 More recently, FMT has been associated with a survival benefit in steroid-resistant alcoholic hepatitis compared with standard of care.79
Bajaj explained that the use of gut-selective broad-spectrum antibiotics have been shown to be equally effective at reducing inflammation in patients with acute alcoholic hepatitis as standard of care.80 Similarly, studies of rifaximin in alcohol-related hepatitis indicate that this antibiotic is associated with lower infection rates and liver-related complications.81,82 Bajaj pointed out that these data suggest that antibiotics may have some kind of impact on liver-related outcomes, but that larger clinical trials are needed.
Although FMT appears promising as a treatment for liver disease, Bajaj emphasised that achieving regulatory approval for such treatments is a significant challenge due to inherent variation in human stool samples.83 One alternative approach to microbiome modification is the use of bacteriophages. In animal models, the use of this technique to reduce the levels of cytolysin-producing E. faecalis in the gut has shown promise in alcohol-related hepatitis.5
HEPATITIS B-RELATED LIVER DISEASE
Altered Faecal Microbiome and Metabolome in Hepatitis B-Related Chronic Liver Diseases
Yue Shen
Shen presented the results of a microbiome and metabolome analysis study to characterise the gut microbiota in patients with HBV-CLD (Shen Y et al., ILC 2022, unpublished data).
Faecal samples from 64 patients with HBV-CLD and 17 healthy controls were submitted for 16S ribosomal RNA sequencing. Faecal metabolomics were measured in a subgroup of 58 subjects, and changes in peripheral blood mononuclear cells were assessed following exposure to bacterial extracts from patients with HBV-CLD and controls (Shen Y et al., ILC 2022, unpublished data).
Results showed that faecal microbiota and metabolites of patients with HBV-CLD differed from those of healthy controls (p<0.01), and these differences were driven by both severity of disease and antiviral treatment. In patients with cirrhosis, the relative abundance of Faecalibacterium was higher, and Turicibacter and Adlercreutzia were lower compared with patients without cirrhosis and healthy controls. In addition, the abundance of Blautia, Dorea, and ruminococcaceae UCG-013 was similar between healthy controls and patients treated with antiviral, but were significantly lower in patients who were not treated, suggesting that antiviral treatment may be able to correct the gut dysbiosis associated with these species in HBV-CLD. A similar trend was seen with Fusicatenibacter, Eubacterium hallii, and Anaerostipes (Shen Y et al., ILC 2022, unpublished data).
Metabolomic profiling revealed decreases in 12-ketodeoxycholic acid and isobutyryl-L-carnitine and increases in 20-hydoxy-leukotriene E4 and 2-ethyl-2-hydroxybutyric acid in patients with cirrhosis compared with patients without cirrhosis and healthy controls. Metabolites involved in histidine metabolism were also found to be increased in patients with cirrhosis versus healthy controls. In terms of antiviral treatment, deoxycholic acid, capric acid, and sebacic acid levels were lower, and faecal phosphatidylcholine higher, in untreated patients compared with both healthy controls and patients treated with antiviral (Shen Y et al., ILC 2022, unpublished data).
Exposing peripheral blood mononuclear cells to bacterial extracts showed that extracts from HBV-CLD patients attenuated the expansion of T helper (Th) 1 cells (p=0.002) and promoted the expansion of Th17 cells (p=0.004) compared with extracts from healthy controls. Bacterial extracts from patients with cirrhosis appeared to have the most impact on Th1 cells, whereas extracts from patients without cirrhosis appeared to have the most impact on Th17 cells.
Shen concluded that both disease progression and antiviral treatment contributed to a compositional shift of gut microbiota and metabolites in patients with HBV-CLD. She suggested that bacterial products may impact the prognosis of patients with HBV-CLD through peripheral immune cells.
HEPATIC ENCEPHALOPATHY
New Gut-Based Approaches to Therapy in Hepatic Encephalopathy
Jasmohan S. Bajaj
Bajaj emphasised that gut microbiota are critical to the development of HE, and can be used as diagnostic and/or prognostic markers (Bajaj JS et al., ILC 2022, unpublished data). He explained that, theoretically, gut-based therapies have treatment potential for HE; but that there are challenges associated with these approaches.84 He reviewed a number of therapies.
Lactulose is a relatively inexpensive gut-based therapy that increases the frequency of bowel movements and is used to prevent overt HE recurrence. However, it induces minimal changes in microbiome structure and function, is sometimes poorly tolerated, and is associated with poor adherence and acceptance rates, particularly in Western countries.85-87
Rifaximin has been shown to improve cognitive function, reduce HE episodes, and modify gut microbial structure and function.10 Yet, Bajaj explained, rifaximin requires bile in order to solubilise for optimal bioavailability,88 and this can be an issue in patients with cirrhosis. A new formulation of rifaximin (soluble solid dispersion) has recently been developed to solubilise in water, without the need for bile acids. This formulation reduced mortality or hospitalisation in outpatients with controlled ascites compared with placebo. In combination with lactulose, it accelerated the resolution of confusion in patients with overt HE compared with lactulose alone.89 Based on these findings, rifaximin soluble solid dispersion is being studied in a Phase III clinical trial (RED-C) to determine efficacy in reducing early decompensation in cirrhosis (NCT05071716).90
Bajaj explained that FMT therapy been shown to be well-tolerated and to have promising efficacy in HE, and there are currently two ongoing clinical trials of FMT in this therapy area: THEMATIC, a trial of FMT in patients with cirrhosis and HE; and PROMISE, a trial of FMT in patients with cirrhosis. As an alternative to crude FMT, rationally defined bacterial consortia are also being tested as gut-based therapeutic approaches in HE, including a group of eight bacterial strains selected for their ability to resist Clostridium difficile colonisation (VE-303; NCT03788434),91 and the use of preserved, lyophilised, and encapsulated FMT (RBX7455; NCT02981316).92