Abstract
Objective: The primary objective was to compare the risk factors and trends of patients with COVID-19 with and without biochemical evidence of myocardial injury. Secondary objectives were the duration of hospitalisation, admission to an intensive care unit, mode of resuscitation, and use of supplemental O2.
Design, and place and duration of study: This retrospective cohort study was conducted at Shifa International Hospitals, Islamabad, Pakistan. The study was conducted from May 2021–May 2022.
Methodology: 113 patients admitted with a confirmed diagnosis of COVID-19 and troponin-I investigation were included in the study. Patients were divided into two groups: with and without myocardial injury on admission. Demographics, symptoms, diagnostics, vitals, comorbidities, and outcomes were noted. The χ2 exact test for categorical variables and independent t-test for continuous variables were used. All statistical analyses were performed with SPSS Statistics®, version 26.0 (IBM®, Armonk, New York, USA), with 0.05 as a level of significance.
Results: Out of 113 patients, 47 patients (42%) had no myocardial injury on admission. The mean age of the patients was 63 years. Patients with myocardial injury were older, male, and unvaccinated against COVID-19 (p<0.01). No vital signs or presenting symptoms were statistically significant for myocardial injury. A comorbidity of hypertension on admission was suggestive of myocardial damage. Patients with myocardial injury had a higher risk of requiring resuscitation by cardiopulmonary resuscitation or pharmacologic means.
Conclusion: Patients with myocardial injury did not have any impact on the composite of secondary outcomes, such as the duration of hospitalisation, admission to an intensive care unit, and use of supplemental O2.
Key Points
1. Acute myocardial injury (AMI) occurred in 0.5% of patients who had recovered from COVID-19, based on a large population of more than 20 million patients. Additionally, after COVID-19 recovery, survivors’ risk of AMI increases by almost 93%. Hence, it is pivotal to understand the link between myocardial injury and COVID-19 disease.
2. The authors present a prospective observational cohort study on 113 patients with COVID-19.
3. When treating patients with COVID-19, the increased risk of myocardial injury necessitates a multifaceted strategy, which includes early detection, vigilant monitoring, and specialised therapies. Medical practitioners need to be on the lookout for cardiac involvement in patients with COVID-19, particularly troponin elevation, and take swift action to prevent future consequences.
INTRODUCTION
Coronavirus disease is a respiratory infection caused by the severe acute respiratory syndrome coronavirus 2 (SARS-CoV-2) coronavirus that has resulted in a worldwide pandemic.1 Coronaviruses are positive-sense, single-stranded RNA viruses with particles of irregular shape, and a crown-like outer covering composed of club-shaped polymers. Its strains have been implicated in respiratory illness in the past, both in rare cases of immunocompromised individuals, and in community-based outbreaks.2,3 Following COVID-19, there has been growing interest in the impact of viruses on the cardiovascular system, even though they have long been implicated in a host of cardiovascular complications, such as myocarditis, acute myocardial infarction, and exacerbation of heart failure.4-6 The spike protein for SARS-CoV-2 is important in its transmission and pathophysiology, particularly in respiratory illness, but it can also infect cardiac tissue by binding to angiotensin-converting enzyme-2 (ACE-2) receptors, causing inflammation and myocardial damage. Animal models and autopsies of the heart have demonstrated SARS-CoV-induced damage to the myocardium.7-10
Myocardial infiltration by mononuclear cells and lymphocytes has also been seen in patients with COVID-19.11-13 There are a myriad of cardiovascular complications after COVID-19 infection, such as myocardial injury, acute myocardial infarction, myocarditis, endocardial fibrosis, venous thromboembolism, and arrhythmias cited in the literature.14 These complications are linked to pre-existing comorbidities, such as diabetes; pre-existing cardiovascular disease; renal disease; pulmonary disease; coagulation abnormalities; and deranged laboratory parameters, such as elevated C-reactive protein (CRP), troponin, and deteriorating left ventricular ejection fraction (EF).15,16
Patients with underlying illnesses are at a greater risk for complications or death; according to early COVID-19 case reports, up to 50% of hospitalised patients have underlying comorbidity. In the largest reported clinical cohort of COVID-19 to date,17 acute cardiac injury, shock, and arrhythmia were observed in 7.2%, 8.7%, and 16.7% of patients, respectively. Cardiac troponin is the recommended biomarker for myocardial necrosis, and it is a key part of diagnosing myocardial infarction.18-20 The creation of more sensitive assays has enhanced our ability to quantify cardiac troponin, with the new generation of high-sensitivity assays capable of detecting cardiac troponin concentrations in the majority of healthy individuals.21 This has allowed us to understand how baseline cardiac troponin levels can serve as prognostic indicators in populations that are at risk of cardiovascular disease, and has opened the door for further research in that domain.22
To this day, information regarding the effects of coronavirus on the myocardium and the mortality related to this is insufficient. It is important to know which patients have a higher chance of developing myocardial damage. This study aims to provide data on patients with COVID-19, with and without myocardial disease, to physicians in order to help tailor patient care.
MATERIALS AND METHODS
This single-centre retrospective study was conducted at Shifa International Hospital, Islamabad, Pakistan, from March 2021–May 2022. Patients were sampled using the consecutive sampling method. All patients 12 years of age and above admitted to the authors’ hospital with a reverse transcriptase-PCR confirmed diagnosis of COVID-19 were included in the study. Patients who did not require admission were excluded from the study. The study was approved by the Institutional Review Board and Ethics Committee of Shifa International Hospital, reference number 352-21.
The authors collected data from 113 subjects using non-electronic and electronic medical record data, including progress reports, graphic charts, laboratory reports, and imaging data. Patients were divided into two groups: with and without myocardial injury on admission, defined as high-sensitivity cardiac troponin I (cTnI) levels >34.4 pg/mL in males, and >15.6 pg/mL in females. Various parameters were reported on a web-based questionnaire. Data collected included age, sex, BMI, presenting symptoms, diagnostics, vitals, and comorbidities. Patients with raised troponin were also asked about ischaemic symptoms, such as jaw pain, chest pain, and shortness of breath. Vaccination against COVID-19 was also noted.
All patients had an extensive work-up done upon admission, which included a complete blood count; liver function tests; renal function tests; serum electrolytes; coagulation profiles, such as activated partial thromboplastin time; and cardiac and inflammatory biomarkers, such as cTnI, CRP, procalcitonin, lactate dehydrogenase (LDH), ferritin, and D-dimers. All patients underwent high-resolution CT (HRCT)/chest X-ray imaging at admission, and findings were recorded. Any rise in cardiac biomarkers prompted transthoracic echocardiogram and electrocardiogram (ECG). All medications used by the patients were documented, as well as the mode of ventilation, use of supplemental O2, length of stay at the hospital, intensive care unit (ICU) admission, mode of resuscitation, and any other co-interventions.
The primary objective was to compare the risk factors and trends of patients with COVID-19 with and without biochemical evidence of myocardial injury. Secondary outcomes were the duration of hospitalisation, admission to ICU, mode of resuscitation, and use of supplemental O2.
Patient and Public Involvement
The authors clarify that patients were not involved in this research.
Statistical Analysis
Categorical variables are presented as percentages, and continuous variables as mean and standard deviation. Categorical variables are sex, symptoms, comorbidities, mode of confirming the diagnosis, ECG pattern, echocardiographic findings, medication, interventions, mode of resuscitation, ICU stay, lung infiltrates on chest X-ray/CT, prior vaccination against COVID-19, and clinical events during hospitalisation.
Continuous variables are age; weight; height; BMI; vital signs, including lowest blood pressure reading, highest respiratory rate, lowest saturation, and highest pulse recorded; troponin; LDH; ferritin; D-dimer; complete blood count; electrolytes; CRP; creatinine phosphokinase; creatine kinase-myocardial band (CKMB), serum urea; liver function tests; and length of hospital stay. The χ2 exact test for categorical variables and independent t-test for continuous variables were used. All statistical analyses were performed with SPSS® Statistics, version 26.0 (IBM®, Armonk, New York, USA), with 0.05 as a level of significance.
RESULTS
A total of 1,375 patients presented to the authors’ centre with reverse transcriptase PCR-confirmed coronavirus disease, out of which 1,215 were managed on an outpatient basis and were excluded. A total of 160 patients with reverse transcriptase-PCR-confirmed COVID-19 were admitted, out of which 113 patients had a high sensitivity troponin-I test at baseline, and were enrolled in the study. Table 1 shows the baseline characteristics of the patients. Patients were divided into two groups: Group A, which included patients without myocardial injury, and Group B, which included patients with myocardial injury on admission. The mean high sensitivity troponin-I was 1115±5625 pg/mL. Out of 113 patients, 47 patients (42%) had no myocardial injury on admission. The mean age of the patients was noted to be 63 years, which was higher for those with myocardial injury compared with those without myocardial injury (67 versus 61; p<0.01). Patients with myocardial injury were older, male, and unvaccinated against COVID-19 (p<0.01). No vital signs were statistically significant for myocardial injury. All 113 patients had a chest X-ray at the time of admission, of which 112 (99.3%) patients had a COVID-19-like picture, and one (0.7%) did not. HRCT was done for 101 individuals, of which 89 (86.4%) patients had a COVID-19-like picture, whereas 12 (11.7%) did not. HRCT could not be performed for two (1.9%) patients.
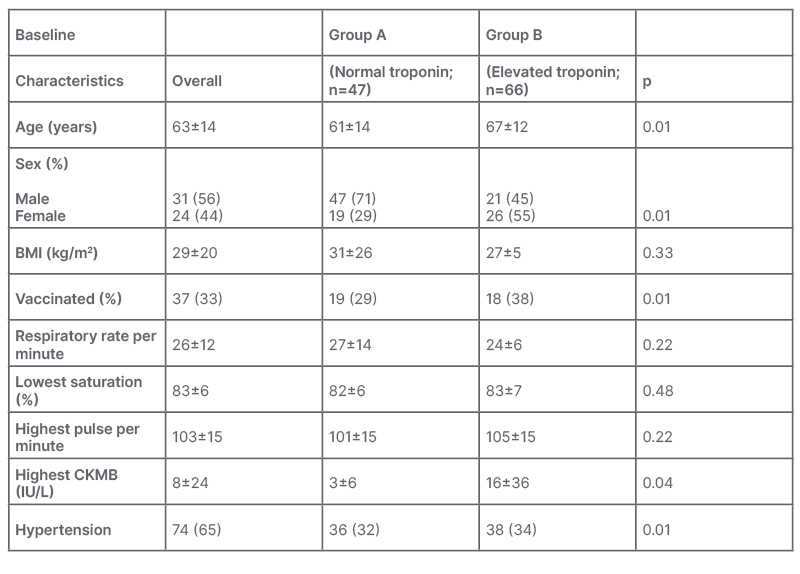
Table 1: Baseline characteristics of both groups.
CKMB: creatine kinase-myocardial band.
The patients presented with a host of signs and symptoms. The most common symptom was fever (88%), followed by shortness of breath (81%), cough (69%), and myalgia (56%). Only 2% of the patients were asymptomatic. No symptom was statistically significant for myocardial injury (Figure 1A).
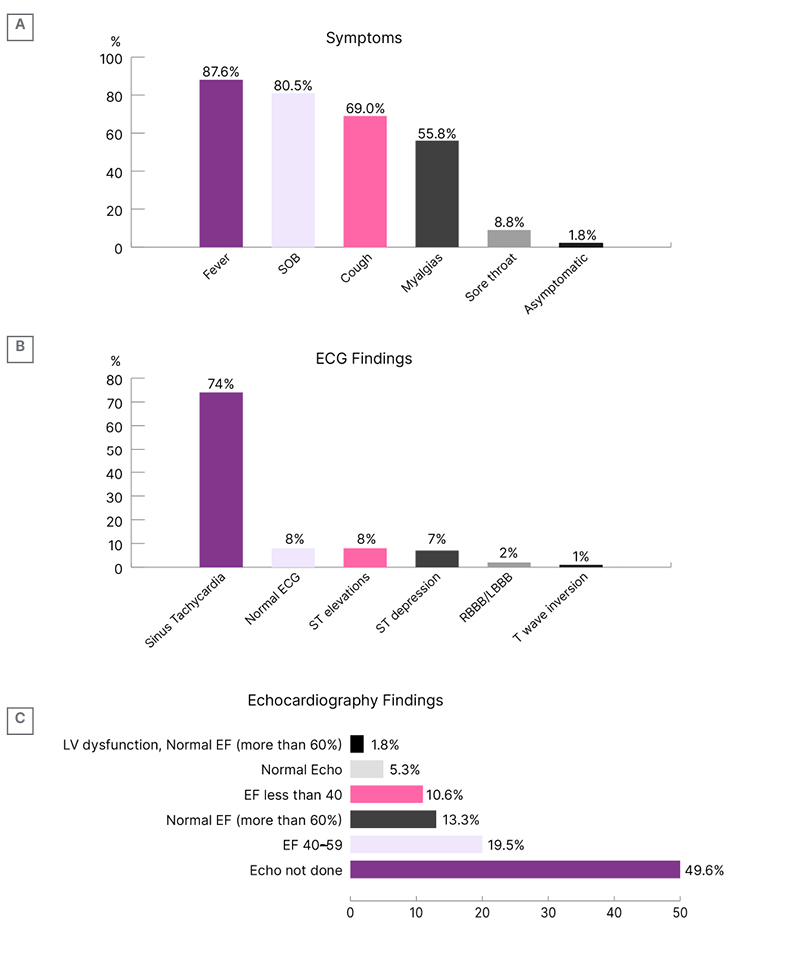
Figure 1: Clinical picture of the study population.
A) Presenting symptoms of the patients. B) Electrocardiography findings of the patients. C) Echocardiography findings of the patients.
ECG: electrocardiogram; EF: ejection fraction; LBBB: left bundle branch block; LV: left ventricle; SOB: shortness of breath; RBBB: right bundle branch block.
The laboratory differences and baseline characteristics between the two groups, elevated troponin (n=47) and normal troponin (n=66), were compared. Out of all parameters in the authors’ study, a raised CKMB and comorbidity of hypertension were found to be statistically significant for myocardial injury. This is in line with the normal diagnostic approach to myocardial injury, specifically in the case of myocardial infarction.23 The parameters measured include CKMB (IU/L), serum ferritin (µg/L), CRP (mg/L), LDH (units/L), and white blood cell count (109). The mean value and standard deviation of each parameter were obtained for both groups. The p value, which indicated the level of statistical significance between the two groups, was also identified. For CKMB, the mean value for the elevated troponin group (8±24) was higher than the normal troponin group (3±6), with a p value of 0.04, which was considered to be statistically significant at a level of 0.05. The mean value of serum ferritin in the elevated group (1163±1472) was slightly higher than in the normal group (1058±1349), with a p value of 0.99, indicating no statistical significance. In a similar vein, the elevated group’s mean CRP (76) was higher than the normal group (57), with a p value of 0.18, indicating no statistical significance. The mean value of LDH in the elevated group (459±251) was lower than in the normal group (686±417), with a p value of 0.59, indicating no statistical significance. Similarly, the mean value of white blood cell count in the elevated group (16938±16848) was lower than in the normal group (15681±3777), with a p value of 0.52, again indicating no statistical significance. In conclusion, the only parameters that showed a statistically significant difference between the two groups were CKMB level and comorbidity of hypertension, with a p value of less than 0.05.
In the authors’ study of 113 patients and their associated comorbidities, 74 (65%) had hypertension, highlighting it as the most common comorbidity. This was followed by 55 (49%) patients with diabetes, 29 (26%) with ischaemic heart disease, 12 (11%) with chronic kidney disease (CKD), nine (8%) with chronic lung disease, seven (6%) with malignancy, and three (3%) with a stroke. Additionally, one patient (1%) each had heart failure and chronic liver disease. Of the 47 patients with elevated troponin levels, 38 (34%) had hypertension, 27 (24%) had diabetes, 14 (12%) had ischaemic heart disease, eight (7%) had CKD, five (4%) had chronic lung disease, three (3%) had malignancy, two (2%) had a stroke, and one (1%) had heart failure. Of the 66 patients with normal troponin levels, 36 (32%) had hypertension, 28 (25%) had diabetes, 15 (13%) had ischaemic heart disease, four (3%) had CKD, four (3%) had chronic lung disease, four (3%) had malignancy, one (1%) had a stroke, and none had heart failure or chronic liver disease. The difference in hypertension between the two groups was significant (p=0.01), while the difference in CKD approached significance (p=0.06). The differences in other comorbidities were not found to be significant.
Both groups of patients had ECG and echocardiogram done at some point during their hospital stay. The most common finding on the ECG was sinus tachycardia (74%), followed by ST elevation (8%). Eight percent of patients had normal ECG (Figure 1B). Findings on the echocardiography report were stratified according to EF. Most patients (20%) had an EF of 40–59% (Figure 1C).
The length of hospital stay ranged from 4–25 days, with an average of 9 days. Overall, a total of 22 (20%) patients required ICU admission, which was equal for both groups. Mechanical ventilation was used in nine (8%) patients, which was more for patients with myocardial injury, but this value was not statistically significant. A total of 14 patients (12%) developed myocardial infarction, nine patients (8%) developed arrhythmias, and three patients (3%) required revascularisation. One patient (1%) developed non-fatal cardiac arrest, one (1%) patient developed lower limb deep venous thrombosis, one (1%) patient developed arterial thrombus, and one (1%) patient developed acute heart failure. Four patients (3%) required cardiopulmonary resuscitation, and all of these patients had a myocardial injury (p=0.02). Seventeen patients required pharmacologic resuscitation, which was more for patients with myocardial injury. Five patients (4%) died during the hospital stay (Table 2).
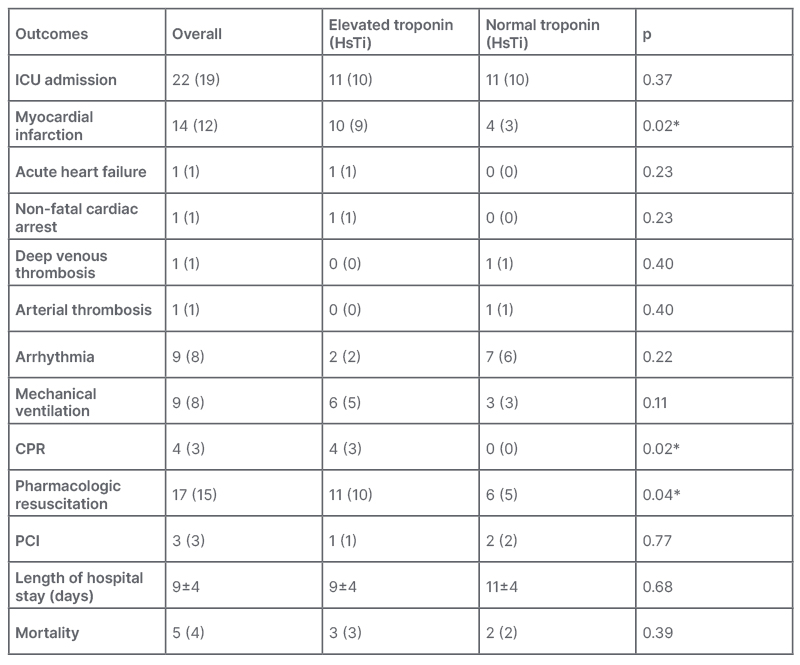
Table 2: Clinical outcomes between both groups.
*Denotes the values are statistically significant.
CPR: cardiopulmonary resuscitation; HsTI: high sensitivity troponin-I; ICU: intensive care unit; PCI: percutaneous coronary intervention.
DISCUSSION
In late 2019, there was an identification and subsequent dissemination of an unfamiliar infectious pneumonia originating from Wuhan, China. The causative agent in question can be classified as SARS-CoV-2, which is commonly referred to as COVID-19.24 The manifestation of symptoms resulting from COVID-19 infection exhibits disease progression that extends beyond the respiratory system, affecting various organ systems. In a previous report, a patient afflicted with SARS-CoV-2 exhibited left ventricular dysfunction and pericarditis in the absence of any respiratory manifestations.25
It is crucial to comprehend the risk factors pertaining to cardiac involvement in COVID-19 infection. Prior studies have indicated a heightened mortality rate in cases where infections are accompanied by cardiac injury.26,27
Acute cardiac injury is a comprehensive phrase that may encompass various cardiovascular conditions. With the accumulation of additional data from a larger patient population, the evidence supporting the correlation between acute cardiac injury and unfavorable outcomes in individuals with COVID-19 is increasingly robust. According to a meta-analysis conducted on COVID-19 data obtained from peer-reviewed publications, it has been observed that the occurrence of acute cardiac injury in individuals diagnosed with COVID-19 was found to be approximately 15%. Furthermore, the analysis revealed that severe cases of COVID-19 were nearly five times more prone to exhibit acute cardiac injury in comparison to milder cases.28
The medical terminology ‘acute (or chronic) myocardial injury’ is used to describe a situation where the levels of cTnI exceed the upper reference limit of the 99th percentile. In the broader populace, elevated troponin levels are correlated with an increased propensity for cardiovascular disease and mortality. Nevertheless, after accounting for additional risk factors, it has been observed that troponin I levels exhibit a correlation with mortality associated with cardiovascular disease, whereas troponin T levels demonstrate a correlation with mortality unrelated to cardiovascular disease. In various published studies concerning Chinese patients diagnosed with COVID-19, it has been observed that a notable proportion of study participants, ranging from 8–12%, exhibited elevated levels of cTnI.29-31
Similar to earlier studies of other viral infections, acute cardiac injury in COVID-19 caused by SARS-CoV-2 infection has been primarily defined as an elevation in serum cardiac markers above the 99th percentile upper reference range. The incidence has ranged from about 8–36%. The mortality rate has been significant, over 50% in the initial reports, when using an expansive and comprehensive definition of acute cardiac injury as an elevated high-sensitivity troponin I or troponin T upon admission, or throughout hospitalisation.32-35
CTnI and cardiac troponin T (cTnT) are sarcomeric proteins that are found in the cardiomyocytes, and have a unique aminoacidic protein structure that is distinct from that of skeletal muscle troponins. When myocardial necrosis (i.e., myocardial injury) occurs, the injured cardiomyocytes release the cardiac troponin. The levels of cTnI and cTnT are measured and quantified in the blood plasma to assess for cardiac injury.36,37 Specifically for COVID-19, patients diagnosed with it frequently display acute cardiac injury, which is defined as an increase in the levels of high-sensitivity cTnI or cTnT during their hospital stay, or at the time of admission. The prevalence of this condition varies between 10–35%, depending on the population being studied and the method of measurement.38 Further clarity is still required with regard to the mechanism that culminates in myocardial injury, either singularly or synergistically. The pathological response of the virus itself has been attributed to SARS-CoV-2 adhering to the ACE-2, a membrane-bound protein, to enter respiratory and cardiac cells. Although the affinity for ACE-2 in SARS-CoV-2 is lower than that shown by SARS-CoV-2, and the former has displayed less associated cardiac pathologies, a comparable pattern is still seen. It was observed that, despite ACE-2 receptors being present on vascular endothelial cells, SARS-CoV-2 activity was not found on them. As a result, other paradigms need to be carefully considered.39-43
Other hypothesised pathways of myocardial injury include a cytokine storm driven by an inappropriate response by Type 1 and Type 2 T helper cells and COVID-19 infection-induced hypoxemia, and respiratory failure, causing damage to the myocardial cells.44 As described in the literature, an increase in viral load leads to a rise in cytokine expression, which fuels the inflammatory response.45 Another study examined 95 autopsies of patients who were SARS-CoV-2-positive, and discovered that the virus targeted endothelial cells more specifically, which they hypothesised could further destroy myocytes through paracrine effects.46 They also discovered that increased transcriptomic changes were seen, which can heighten the immune response and exacerbate pro-inflammatory gene expression.46 The burden of inflammation and endothelial dysfunction can also drive the pathogenesis of heart failure, particularly in cases of SAR-CoV-2-induced acute myocarditis.47
COVID-19 infection-induced myocarditis has also been described in the literature; however, the pathogenesis is still not understood, especially as some cases of myocarditis exhibit direct myocyte injury and some do not. Various case reports describe acute myocarditis in the setting of COVID-19, and while myocardial involvement is involved in a worse prognosis, such has not been established for isolated myocarditis.48 However, the fatality increases when both acute respiratory distress syndrome and cardiac inflammation are present; thus, the probability of myocarditis should be taken into consideration when making the appropriate diagnosis based on the clinical and laboratory data.49
The authors’ findings were statistically significant for raised CKMB in the elevated troponin group, signifying myocardial injury. This relationship has already been established in the literature, and only serves to reinforce the presence of acute myocardial damage.50 Additionally, Han et al.51 demonstrated that raised venous CKMB levels and cTnI levels in COVID-19 infection are associated with increased severity and case fatality.
Amongst both groups, 74% had sinus tachycardia on ECG, a pattern that has been observed and described before as the most common electrocardiac abnormality seen in COVID-19 infection.52 A similar trend was seen in a study conducted on patients presenting with severe acute respiratory syndrome, where 71.9% of the patients had sinus tachycardia at some point of hospitalisation. Clarity is needed if this is reference to the normal physiological response, or specific to the virus species.53 However, the presence of persistent tachycardia has also been seen in the manifestation of post-acute COVID-19 syndrome, indicating possible lasting cardiovascular effects of the viral infection.54 The phenomenon has been attributed to heart tissue inflammation, particularly myocardial inflammation, which is as widespread as 78% in patients who are post-COVID-19 infection, and causes tachycardia, palpitations, and chest pain in the months following discharge.55,56
In the authors’ study, 8% of patients demonstrated ST-elevations on ECG, 7% had ST depression, and 1% had T-wave inversions, which are in line with the current literature that describes myocardial injury in COVID-19. These ECG patterns have been mostly described in patients with COVID-19 admitted to ICU.57 An additional study revealed that 41% of patients displayed non-specific repolarisation changes, such as ST segment and T-wave abnormalities. This finding was reportedly the result of myocardial injury, and was linked to negative outcomes, such as a greater need for ICU admission, more frequent use of mechanical ventilation support, and higher mortality.58,59 ST-elevations seem to be a rare presentation, as demonstrated by the literature,60,61 but additional clarity is required to distinguish ST-elevation aetiology in COVID-19 infection.
Tavazzi et al. were the first to describe acute myocardial injury due to COVID-19. The patient reported flu-like symptoms, respiratory distress, hypotension, and cardiogenic shock. The patient was treated with venous-arterial extracorporeal membrane oxygenation and mechanical ventilation, and had an uneventful recovery. Viruses were found in the myocardium, suggesting either a viraemic phase, or the migration of infected macrophages from the lungs into the heart.13
Cardiovascular complications after COVID-19 infection have been sporadically reported in the literature. According to the preliminary results of the LATE-COVID study by Lewek et al.,15 severe cardiovascular complications were observed in 27.5% of inpatients. In comparison to those with mild complications, patients with severe complications had a significantly higher prevalence of diabetes, a decreased EF, higher resting heart rate at admission, and higher levels of CRP and troponin T. Dyspnoea and exercise intolerance were also more frequent in patients with severe complications. These findings were limited to a small cohort of 51 patients, but directed attention toward the possible cardiovascular implications of COVID-19.15
A study by Ferrante et al.62 explored the risk factors for myocardial injury, using a chest CT scan as the screening test. The study included 332 patients, of whom 37% had a myocardial injury, which was lower than the authors’ value of 58.1%. Despite the higher myocardial injury ratio in the authors’ cohort, the authors’ mortality rate was much lower (4.4% versus 20.5%). Ferrante et al.’s62 findings concluded that myocardial injury was independently associated with an increased risk of death. Older age, lower estimated glomerular filtration rate, and lower pressure of O2in blood/fraction of inspired O2 ratio on admission were other independent predictors for both myocardial injury and death. The main risk factors in the authors’ study were old age, male sex, high CKMB on admission, and the comorbidity of hypertension. CKD was found more in patients with myocardial injury in the authors’ study, but the results were not statistically significant.62
Guo et al.34 studied 187 patients with COVID-19-associated cardiovascular complications, out of whom 23% died. The mortality, again, was higher than that reported in the authors’ study. The mean age of their cohort was 58.50 years, which was lower than in this study (63.38 years). Subsequently, they had a lower percentage of myocardial injury (27.8%). Overall, 91.2% of the authors’ cohort had pre-existing cardiovascular comorbidities, such as hypertension and ischaemic heart disease. This was higher than that reported by Guo et al.,34 who reported 35.3% of patients.
A meta-analysis by Yang et al.63 revealed the most common comorbidity in patients with COVID-19 to be hypertension, which was 21.1% in their study and 65.5% in the authors’ study. The stark difference could be due to the higher rate of hypertension in Southeast Asian populations. Diabetes and ischaemic heart disease were the second and third most common comorbidities in their study, as well as in the authors’; however, the authors’ percentage of comorbidities was higher. This skew in the data could be because more patients with comorbidities prefer admission to the authors’ hospital, as it is privately run.
LIMITATIONS
The authors’ study had a few limitations. Their results were based on a small cohort, which cannot extrapolate findings to a larger population. The authors also did not have data regarding myocardial injury before admission, to rule out previous/chronic myocardial injury, which may confound the results of troponin testing.
Findings from a single-centre study with a limited sample size may not be representative of the broader population or different healthcare settings. These study results might be influenced by confounding factors, such as patient demographics, healthcare practices, and resource availability, which could limit the generalisability of the authors’ findings to other regions or healthcare systems.
Differences in laboratory techniques, equipment, or personnel expertise could have introduced measurement bias and affected the reliability and validity of the results.
The determination of secondary outcomes, such as the duration of hospitalisation, admission to ICU, mode of resuscitation, and use of supplemental O2, might have been subjective or influenced by factors, such as healthcare provider preferences or availability of resources. This subjectivity could have introduced bias into the assessment of these outcomes.
The study’s duration and timing of data collection might have limited the ability to capture the full spectrum of COVID-19 outcomes. The pandemic was dynamic, and the course of the disease, as well as treatment strategies and guidelines, evolve over time. Therefore, a single-centre study might not capture the latest trends, or provide insights into the long-term outcomes of patients with COVID-19, and this could be a possible temporal limitation of the study.
CONCLUSIONS
Acute myocardial injury, as indicated by troponin elevation, is common in patients with COVID-19, particularly in the elderly with underlying comorbidities. It is associated with increased risk of progressive severe disease, myocardial infarction, need for cardiopulmonary resuscitation, and pharmacologic resuscitation. Addressing the increased risk of myocardial injury in patients with COVID-19 demands a multifaceted approach that encompasses early detection, close monitoring, and tailored interventions. Healthcare professionals should remain vigilant in recognising signs of cardiac involvement in patients with COVID-19, including troponin elevation, and promptly implement appropriate measures to mitigate further complications. Therefore, targeted treatment and preventative strategies are urgently needed for this vulnerable patient group.