Abstract
In the search to develop new cardioprotective therapies, considerable interest has focussed on approaches for targeting the biological functions of high-density lipoproteins (HDL). This is based on data from population and animal studies demonstrating a potentially protective impact of HDL on cardiovascular risk. The findings of recent clinical trials of a range of therapeutic interventions aimed at promoting HDL have been disappointing and raise considerable uncertainty regarding the potential utility of this target. More recent evidence has highlighted the importance of HDL functionality, which may ultimately be important in terms of its association with cardiovascular risk. This has led to ongoing efforts to develop new risk markers and therapeutics focussing on HDL quality as opposed to quantity. The evidence supporting a protective role for HDL and findings of clinical trials of HDL-targeted therapies are reviewed here.
INTRODUCTION
For >20 years, clinical trials have consistently demonstrated that the reduction of levels of low-density lipoprotein (LDL) cholesterol results in lower cardiovascular event rates, in both the primary and secondary prevention setting.1 While guidelines for cardiovascular prevention have promoted widespread use of LDL cholesterol-lowering agents for patients at high vascular risk, many continue to experience clinical events.2This residual risk highlights the need to develop additional strategies to achieve a more effective reduction of cardiovascular risk in patients with atherosclerotic cardiovascular disease.
EVIDENCE SUPPORTING A PROTECTIVE ROLE OF HIGH-DENSITY LIPOPROTEINS
Following the early evidence that patients admitted to the coronary care unit with myocardial infarction had lower levels of high-density lipoprotein (HDL) cholesterol, large population studies demonstrated an inverse association between HDL cholesterol levels and prospective cardiovascular risk.3-6 Similar findings were observed in patients achieving very low LDL cholesterol levels, in which low HDL cholesterol continued to be associated with higher rates of cardiovascular events.4 Animal studies demonstrated that promoting HDL functionality, via direct infusion or transgenic expression of its major proteins, had a favourable impact on both the burden and composition of atherosclerotic plaque.7-12 Functional studies revealing that HDL exert favourable effects on inflammatory, oxidative, thrombotic, and apoptotic pathways, in addition to its well-characterised role in reverse cholesterol transport, are likely to underscore its impact on plaque.9,13-16
ESTABLISHED LIPID-MODIFYING STRATEGIES AND HIGH-DENSITY LIPOPROTEINS
Changes in lifestyle factors, including weight loss, accompanied by a reduction in abdominal adiposity and moderate alcohol consumption, have been reported to result in modest increases in HDL cholesterol.17 While statin therapy can raise HDL cholesterol by 3–15%, in clinical trials this has been reported to be independently associated with favourable effects of statins on both plaque progression and cardiovascular events.18,19Fibrates raise HDL cholesterol by 5–20%, showing variable effects on cardiovascular events in multiple clinical trials.20-22 Where a clinical benefit was observed, this was associated with an increase in small HDL particle concentration but not HDL cholesterol concentration overall.23 Niacin is the most effective HDL cholesterol-raising agent currently used in clinical practice. While early studies using immediate-release formulations of niacin reported favourable effects on both angiographic disease and cardiovascular events,24 more recent clinical trials of sustained formulations failed to demonstrate reductions in risk in statin-treated patients.25,26Niacin can prove challenging for patients because many experience the side effect of flushing. Efforts to administer niacin in combination with a prostanoid receptor antagonist reduced the rate of flushing, but did not produce clinical benefit.25,26 Clinical development programmes, accordingly, have sought to develop more effective approaches for targeting HDL (Table 1).
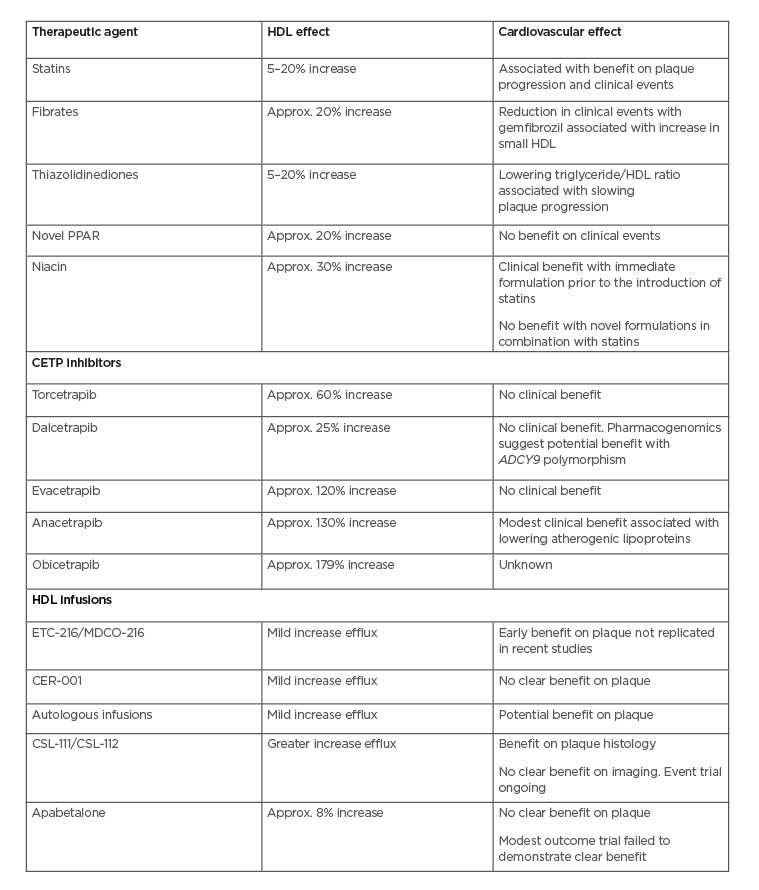
Table 1: High-density lipoprotein-targeted therapies and cardiovascular effects in trials.
Approx.: approximately; CETP: cholesteryl ester transfer protein; HDL: high-density lipoprotein.
HIGH-DENSITY LIPOPROTEIN INFUSIONS
On the basis of favourable effects on atherosclerosis and in-stent restenosis with infusions of reconstituted HDL,7-9,12 interest has focussed on the potential benefits of this approach in humans. Early mechanistic studies in humans demonstrated that HDL infusions increased faecal sterol excretion, a surrogate measure of reverse cholesterol transport, and improved endothelial function.27,28A number of small clinical trials employed serial vascular imaging to evaluate the impact of various formulations of delipidated HDL on atherosclerotic plaque. The first study demonstrated that administration of five weekly intravenous infusions of a HDL mimetic containing recombinant ApoA-I Milano (previously known as ETC-216, now known as MDCO-216) promoted rapid regression of coronary atherosclerosis in patients following an acute coronary syndrome.29 This provided a biological rationale to support observations that humans carrying ApoA-I Milano demonstrated a greater likelihood of longevity and protection from cardiovascular disease.30 It also reaffirmed findings from preclinical studies, demonstrating atheroprotective properties of ApoA-I Milano.31-33 The finding of regression at both 15 and 45 mg/kg doses in the human imaging study suggested a potential saturation effect of this mimetic on lipid transport out of the vessel wall.29
A second clinical development programme of a HDL mimetic, a combination of wild-type ApoA-I and a phospholipid (CSL-111), demonstrated a reduction in plaque lipid and macrophage content when administered 5–7 days prior to femoral endarterectomy.34 A subsequent coronary imaging study in patients following an acute coronary syndrome reported a non-significant trend towards plaque regression and an improvement in plaque echogenicity, suggesting potentially favourable effects on plaque stability.35 The potential for both mimetics, with different forms of ApoA-I, to exert beneficial effects supported the potential of delipidated HDL, as opposed to specific properties related to ApoA-I Milano. This was reaffirmed by the report that selective delipidation of a patient’s HDL, followed by autologous infusion, as similarly associated with plaque regression.36
Synthesis of HDL mimetics, in quantities sufficient for human use, has proven to be a challenge and has required refinement of the manufacturing process to produce purified mimetics with a low potential for toxicity. This has led to a second generation of clinical studies which evaluate the impact of HDL mimetics on a background of more intensive lipid-lowering therapy. The findings to date have been variable. A repeat coronary imaging study of the mimetic containing ApoA-I Milano failed to demonstrate plaque regression.37-39 Another mimetic, which contains recombinant ApoA-I and sphingomyelin (CER-001), has been evaluated in numerous imaging-based trials. While the first study failed to demonstrate a favourable benefit for plaque burden,40 a post hoc analysis revealed regression in those treated with the lowest dose (3 mg/kg) and in patients with the greatest burden of plaque at baseline.41 This observation was further tested; however, 10 weekly infusions failed to be beneficial.42 Similarly, early observations of the potential benefit of CER-001 on carotid plaque volume and inflammatory activity failed to be replicated in prospective, randomised clinical trials.43
Despite the disappointing results of these trials, leading to cessation of the MDCO-216 and CER-001 development programmes, hope remains that other HDL mimetics may produce cardiovascular benefit. Refined manufacturing to produce CSL-112 has progressed to clinical evaluation. A large safety study of this formulation revealed no adverse clinical effects of any concern and a substantial increase in ex vivocholesterol efflux capacity.44 The potential improvement in lipid-transporting activity is much greater than that observed with other mimetics. Given the reported association between cholesterol efflux activity and protection from adverse cardiovascular outcomes, there remains considerable interest in the development of this mimetic. Accordingly, a large cardiovascular outcomes trial is currently in progress to determine whether administration of four intravenous infusions of CSL-112 will reduce cardiovascular event rates in patients following an acute coronary syndrome. This represents the first definitive attempt to determine whether the infusion of some form of HDL will favourably reduce clinical events.
CHOLESTERYL ESTER TRANSFER PROTEIN INHIBITORS
Cholesteryl ester transfer protein (CETP) facilitates the movement of esterified cholesterol from HDL to very-low-density lipoproteins and LDL in exchange for triglycerides.45 CETP inhibition has received considerable attention, given its potential to substantially raise HDL cholesterol levels. Observational studies have reported an association between CETP activity and cardiovascular risk, supported by findings of genomic investigations.45-48 In animal models, therapeutic lowering of CETP activity, using antisense oligonucleotides, vaccines, and small-molecule inhibitors, has been reported to have a favourable impact on plaque burden.49-52 As a result, multiple development programmes have aimed to develop CETP inhibitors, with the potential to target the residual cardiovascular risk observed in many statin-treated patients.
However, experience to date with this class of agents has proved a challenge. The first CETP inhibitor to reach an advanced stage of development, torcetrapib, increased the risk of cardiovascular events and all-cause mortality in a large clinical outcomes trial.53 In parallel, the ability of torcetrapib to raise HDL cholesterol by >60% and lower LDL cholesterol by 20%, in addition to statin therapy, failed to favourably impact the progression of carotid intima-media thickness and coronary atherosclerosis.54-56 Subsequent studies revealed that torcetrapib possessed off-target effects, including adrenal excretion of aldosterone and cortisol, aortic wall expression of endothelin, and small elevations in blood pressure.53,57,58 Because these changes were observed in murine models, which do not express CETP, they are likely to reflect a torcetrapib-specific effect that is not as a result of CETP inhibition. Accordingly, there remains interest in the development of other CETP inhibitors that lack such toxic effects.
A number of CETP inhibitors continued to progress in development on the basis that they lack torcetrapib-like toxicity. Dalcetrapib is a modest CETP inhibitor and raises HDL cholesterol by approximately 25% with no effect on LDL cholesterol levels.59,60 A large clinical outcomes trial was terminated prematurely because dalcetrapib did not reduce cardiovascular event rates.61 Evacetrapib is a potent CETP inhibitor and raises HDL cholesterol by >120% and lowers LDL cholesterol by 25–30% when administered as either monotherapy or in combination with a statin.62 However, these more potent lipid effects did not translate to clinical benefit, with another outcome trial stopped due to futility.63 Anacetrapib is also a potent CETP inhibitor and raises HDL cholesterol by >130% and lowers LDL cholesterol by 30%.64 A clinical trial with more extended treatment demonstrated a significant, albeit modest, reduction in cardiovascular events when anacetrapib was administered in combination with a statin.65 This clinical benefit correlated with the degree of lowering of atherogenic lipoproteins, as opposed to the raising of HDL cholesterol. While this result provided some clinical validation that CETP inhibition may be cardioprotective, anacetrapib accumulates within adipose tissue66 and has not progressed to clinical use.
A number of observations from these CETP inhibitor studies provide insights on potential clinical utility. All of the trials have demonstrated that CETP inhibitor use was associated with a lower rate of diagnosis of Type 2 diabetes mellitus.67,68 In those patients with diabetes, CETP inhibitor use was associated with an improvement in glycaemic control.67,68 Whether this reflects favourable effects of HDL on pancreatic β-cell function,69,70 or an additional effect of CETP inhibition, remains uncertain. It may also have implications for broader use in patients at higher risk, who have evidence of prediabetes or insulin resistance.
Pharmacogenomic analysis of the dalcetrapib trial demonstrated a potential clinical benefit in patients harbouring a polymorphism of the ADCY9 gene on chromosome 16. Patients with the AA genotype demonstrated a 39% reduction in cardiovascular events with dalcetrapib compared with placebo, while patients with the GG phenotype demonstrated an increase in events.71 This finding was supported by the demonstration of a greater increase in cholesterol efflux activity and lesser rise in C-reactive protein levels with dalcetrapib treatment of patients with the AA polymorphism.71 This has led to a new clinical trial of dalcetrapib, performed exclusively in patients identified to have the AA polymorphism.72 A similar relationship was not observed with either evacetrapib or anacetrapib.73,74 If demonstrated to be effective in a prospective trial, it would appear to reflect a dalcetrapib-specific phenomenon.
Additional genomic analyses and Mendelian randomisation have demonstrated the cardioprotective effect of having low CETP activity and levels.46,47,75 This benefit was associated with reductions in levels of atherogenic lipoproteins, rather than raising HDL cholesterol. This benefit also appears greater in the presence, rather than absence, of HMGCR, the target of statins. If correct, this would suggest CETP inhibitors are more likely to be protective in the absence of concomitant statin therapy.75 Accordingly, any further development of CETP inhibitors might focus more on LDL-lowering capability, as opposed to raising of HDL cholesterol. Obicetrapib is the most potent CETP inhibitor developed to date, with more profound effects on LDL and HDL cholesterol, using much lower doses than other agents. How development of this agent will proceed remains to be determined.
ADDITIONAL HIGH-DENSITY LIPOPROTEIN-TARGETED STRATEGIES
A number of other approaches have been investigated, with regard to their potential impact on HDL and cardiovascular risk. Pharmacological agonists of the PPAR family have modest effects on HDL, with variable impact on clinical outcomes. Fibrates are modest PPAR-α agonists, with modest HDL cholesterol raising capabilities and evidence of cardiovascular benefit in some,76,77 but not all,22,78 clinical trials. Meta-analyses of these trials demonstrated a borderline clinical benefit of widespread fibrate use, but more definitive benefit when used in patients with evidence of hypertriglyceridaemia at baseline.20 Subsequent analysis of the gemfibrozil studies suggested that its cardiovascular benefit may be associated with the observed 21% increase in small HDL particles.23 In a similar fashion, thiazolidinediones are PPAR-γ agonists which mildly raise HDL cholesterol in addition to their primary role in improving insulin sensitivity. The effect of these agents on HDL is likely to contribute to their potential clinical benefit, with evidence that reducing the triglyceride/HDL cholesterol ratio is most strongly associated with the ability of these agents to slow progression of coronary atherosclerosis on serial imaging.79 Attempts to develop more potent PPAR agonists,80 or agents targeting multiple PPAR,81 have had difficulty with either toxicity or a lack of clinical benefit. The recent development of selective PPAR modulators has the potential to derive specific metabolic benefits without the toxicity observed with standard PPAR agents,82 although the clinical benefit of this approach has yet to be established.
Apabetalone is a selective inhibitor of bromodomain and extraterminal proteins: epigenetic regulators of lipoprotein metabolism, inflammation, and vascular calcification.83,84 Early studies of apabetalone focussed primarily on its potential impact on HDL functionality, on the basis of reports of stimulated ApoA-I synthesis and increase in cholesterol efflux capacity in non-human primates.85 Early clinical trials of apabetalone in statin-treated patients demonstrated modest effects on HDL cholesterol levels86 and progression of coronary atherosclerosis87 with short-term treatment. Pooled analyses of these trials demonstrated fewer cardiovascular events in apabetalone-treated patients compared with those who received placebo.87 This led to a moderate-sized clinical outcomes trial, which failed to demonstrate a significant reduction in clinical events with apabetalone when administered in patients with diabetes and low HDL cholesterol levels, following an acute coronary syndrome.88
Additional approaches to targeting HDL have focussed on specific factors implicated in reverse cholesterol transport. Upregulation of ABCA1, a pivotal factor facilitating cholesterol efflux to receptors such as HDL particles, may mobilise lipids without necessarily modulating systemic HDL concentrations.89 LCAT promotes esterification of cholesterol on the surface of HDL particles, which is subsequently stored within the particle core, maintaining a low concentration of free cholesterol and an environment favouring ongoing transfer of lipids to HDL. Pharmacological LCAT agonists are currently undergoing early evaluation in human studies.90,91 Advocates of genetic replacement therapy have proposed that the ability to upregulate hepatic ApoA-I expression may have the potential to increase generation of nascent HDL particles.92 While early proponents of such an approach suggested a potential role in patients with genetically low ApoA-I levels, evolving approaches in gene editing may provide a more widespread therapeutic option in the future.
DYSFUNCTIONAL HIGH-DENSITY LIPOPROTEINS
While the early work in HDL therapeutics focussed on raising HDL cholesterol levels, the poor results of clinical trials and lack of association between genetic polymorphisms regulating HDL cholesterol levels and cardiovascular risk93 have suggested this may not prove useful. In parallel, the potential benefit of HDL infusions, in the absence of raising HDL cholesterol levels and in addition to reports of the association of cholesterol efflux activity with cardiovascular risk,94 highlights the likelihood that HDL functionality may be more important. This is supported by reports of cardiovascular events in patients with very high HDL cholesterol levels95 and by reports that the functional properties of HDL may be impaired in the setting of hyperglycaemia, inflammation, and carbamylation.96-101 HDL circulates as a heterogenous population of particles, varying in size and composition of both protein and lipid. It may be that different sub-particles may possess varying functional activity. The small, lipid-deplete particles, as evidenced in infusion mimetics, are potent promoters of cholesterol efflux activity. Therapeutic increases in small HDL particles were reported to be associated with the clinical benefit of gemfibrozil.23 In contrast, generation of large, cholesterol-rich HDL particles with CETP inhibition, though proposed to potentially impair efflux activity, was never demonstrated. Nevertheless, future approaches to HDL therapeutics might consider targeting specific HDL subgroups. Measures of dysfunctional HDL may also play a role in risk prediction.102Furthermore, it is possible that modulating factors that render HDL dysfunctional may provide a novel approach to therapeutic targeting of HDL in patients at a high risk. This remains an active area of research interest for investigators still working to develop HDL-focussed approaches to reducing cardiovascular risk.
CONCLUSION
Considerable work has tried to enhance potentially protective properties of HDL to reduce the residual cardiovascular risk in statin-treated patients. While raising HDL cholesterol has proven to be a disappointing strategy, ongoing efforts have focussed on targeting the functional activities of HDL. Whether any such strategies are ultimately likely to be of clinical benefit will require more clinical trials. To facilitate this, the field will need to embrace the need to pivot the HDL story as a narrative worth pursuing. Only time will tell whether this will alter the course of cardiovascular risk.