Abstract
Introduction: Dexmedetomidine has been shown to have a myocardial protective effect in off-pump coronary artery bypass patients. However, the same dosage of dexmedetomidine could not elicit a myocardial protective effect in percutaneous coronary intervention patients. The aim of this study was to assess the effect of different doses of dexmedetomidine when used for myocardial protection in percutaneous coronary interventional patients.
Methodology: 240 patients (Group D1, treated with dexmedetomidine [n=80]; Group D2, treated with dexmedetomidine [n=80]; and the control group [C; n=80]) were enrolled in the study. Dexmedetomidine was administered over 15 minutes in the respective doses in Groups D1 and D2 at the start of the procedure, while normal saline was given to patients in Group C. Maintenance of dexmedetomidine/NS was started at 0.5 µg/kg/hour in the groups until 30 minutes post-procedure. Creatine phosphokinase (CPK) and CPK-MB, heart rate (HR), mean blood pressure (MAP), and sedation score were noted at baseline (T0), 6 hours (T1), 12 hours (T2), and 24 hours (T3) after the loading dose.
Results: MAP and HR significantly decreased in D1 and D2 compared to C (p<0.05). None of the patients in D1 had a reduction in MAP <20% and HR <50 bpm; however, 3 patients in D2 had a clinically significant reduction in MAP, and 5 patients had HR <50 bpm. The patients in D2 were more sedated compared to patients in D1 and C. The difference in CPK and CPK-MB was significant at 6 hours, 12 hours, and 24 hours in D2.
Conclusion: Dexmedetomidine 2 µg/kg provides myocardial protection compared to 1 µg/kg, but at the cost of a clinically significant decrease in MAP and HR. Patients who received dexmedetomidine 2 µg/kg were more sedated compared to patients receiving 1 µg/kg, warranting greater care during and post-procedure.
INTRODUCTION
Acute myocardial infarction (MI) is a major cause of death and disability worldwide. Myocardial injury in acute MI is due to both ischaemic as well as reperfusion injury. Reperfusion injury is defined as damage to the tissue caused when blood supply is restored to the ischaemic area after a certain period. A similar type of injury occurs in the myocardium after acute MI is treated with percutaneous coronary intervention (PCI) or fibrinolytic therapy.1-3 This suggests that the process of reperfusion can itself induce cardiomyocyte death, known as myocardial reperfusion injury.
Various mechanisms have been postulated for reperfusion injury. Reperfusion is associated with microvascular injury, particularly due to increased permeability of capillaries and arterioles. Following reperfusion, there is an imbalance between vasoconstrictors and vasodilators; the activated endothelial cells produce more reactive oxygen radicals but less nitric oxide due to a decrease in endothelial nitric oxide synthase and increase in superoxide. Also, there are increased concentrations of vasoconstrictors such as endothelin I and angiotensin II. This imbalance causes an inflammatory response that is partially responsible for the damage caused by reperfusion injury.4
White blood cells are carried to the reperfused area as a result of the restoration of blood flow, which causes a release of inflammatory factors, such as interleukins (IL) and free radicals.5 Inflammatory mediators cause damage to cellular proteins, DNA, and the plasma membrane, which can cause further release of free radicals. These mediators may also act indirectly through redox signalling to initiate apoptosis. White blood cells may also obstruct small capillaries by binding to the endothelium, worsening the ischaemia.5 The ischaemic tissue has a reduced number of free radical scavengers, which results in further tissue damage after reperfusion. Other mechanisms suggested in reperfusion injury include calcium overload and depletion of high energy phosphate stores.6-8 Reperfusion can cause hyperkalaemia,9 arrhythmias,10 and a rise in cardiac enzymes (Troponin I and T and creatinine phosphokinase [CPK]-MB).11 Thus, identification of these conditions can aid in the diagnosis of reperfusion.
A number of strategies have been used to prevent lethal myocardial reperfusion injury in patients undergoing PCI. Mechanical interventions include remote ischaemic preconditioning, therapeutic hypothermia, and therapeutic hyperoxaemia, whereas pharmacological interventions include adenosine, anti-inflammatory agents, atrial natriuretic peptide, atorvastatin, erythropoietin, glucose-insulin-potassium therapy, and sodium nitrite.12 Despite so many available interventions, there is still no effective therapy to prevent myocardial reperfusion injury. A number of new therapeutic strategies thought to have the potential to improve clinical outcomes in patients with acute MI treated with PCI are currently under investigation for preventing myocardial reperfusion injury; one such therapeutic strategy is dexmedetomidine.
Dexmedetomidine is an α2-adrenergic receptor agonist used for conscious sedation. It has a relatively high ratio of α-2:α-1 activity (1620:1) and is considered a more selective α2 receptor agonist, being 8-fold more selective for the α2 receptor than clonidine.13 Its use is increasing both inside and outside operation theatres because of its unique property of providing sedation without causing respiratory depression.14 The recommended dosing for adults is 1 µg/kg over 10 minutes (loading dose for procedural sedation), with doses ranging from 0.2–1.0 µg/kg/hour (maintenance dosing for procedural sedation). Besides being useful as a sedative agent, dexmedetomidine has also been shown to have a protective effect on many organs in various experimental studies.15-17 Dexmedetomidine has been shown to have a protective effect on myocardial ischaemia-reperfusion injury in rats;18,19 however, there is limited literature detailing the effect of dexmedetomidine during ischaemia-reperfusion injury in PCI patients. Dexmedetomidine has also been shown to have a myocardial protective effect in off-pump coronary artery bypass patients.20,21 However, the same dosage of dexmedetomidine could not elicit a myocardial protective effect in PCI patients as far as a decrease in myocardial enzymes was concerned.22
The aim of this study was to assess the effect of different doses of dexmedetomidine when used for myocardial protection in PCI patients. It was hypothesised that a dose of dexmedetomidine higher than that routinely used may elicit a myocardial protective effect in patients undergoing PCI.
METHODOLOGY
After approval from the institutional ethics committee was obtained, a prospective double-blind randomised controlled trial was conducted over 6 months, from February–July 2017, in a tertiary cardiac care institute. In total, 240 patients undergoing elective PCI for single vessel disease were recruited. Patients with an ejection fraction (EF) of <40%, in cardiogenic shock, and undergoing emergency procedures were excluded from the study. The sample size was calculated as per a previous study, keeping the value of α-error as 0.05 and the power of the study as 80%.23 Patients were randomised using a computer-generated randomisation table. The subjects were randomised into Group D1 (n=80), Group D2 (n=80), and a control group (C; n=80). The groups’ dosing regimens were:
- D1: Injection of dexmedetomidine 1 µg/kg was administered over 15 minutes as a loading dose, followed by 0.5 µg/kg/hour as a maintenance infusion.
- D2: Injection of dexmedetomidine 2 µg/kg was administered over 15 minutes as a loading dose, followed by 0.5 µg/kg/hour as a maintenance infusion.
- C: Injection of 0.9% sodium chloride started and maintained at the same rate as for D1 and D2.
An anaesthesia technician not related to the study prepared the drug in an unlabelled syringe and passed it to the on-duty anaesthesiologist. For D1, 200 µg of dexmedetomidine was added to 50 cc normal saline (NS) (4 µg/cc). For D2, 400 µg of dexmedetomidine was added to 50 cc NS (8 μg/cc). For C, the unlabelled syringe only contained NS. The attending anaesthesiologist was blinded to the drug and dose administered and considered each unlabelled syringe as containing dexmedetomidine 4 µg/cc, calculating the volume to be infused via a syringe pump accordingly, with a routine dose of 1 µg/kg bolus followed by maintenance infusion of 0.5 µg/kg/hour. Thus, the infusate was given at the same rate in all three groups as if the patient were being given dexmedetomidine routinely.
Immediately before the procedure, baseline (T0) CPK and CPK-MB was measured; dexmedetomidine 1 µg/kg, dexmedetomidine 2 µg/kg, or 0.9% sodium chloride infusion was then started. After the loading dose, maintenance infusion of dexmedetomidine or NS was started and continued until 30 minutes post-procedure. CPK and CPK-MB were measured at 6 hours (T1), 12 hours (T2), and 24 hours (T3) after the loading dose.
Heart rate (HR), blood pressure (BP), peripheral oxygen saturation, and sedation score were monitored every 5 minutes during the procedure and every 10 minutes post-procedure for 2 hours. Sedation score was assessed as a per modified Ramsay Sedation Scale (RSS), shown in Table 1.
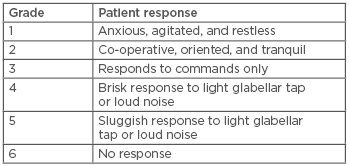
Table 1: Modified Ramsay Sedation Scale.
It was decided to stop the infusion of the unlabelled syringe if mean arterial pressure (MAP) decreased to <20% of baseline or HR decreased to <50 bpm. Rescue drugs, in the form of injected mephentermine in 6 mg boluses intravenously and atropine 0.6 mg injected intravenously, were given for hypotension and bradycardia, respectively. In cases of persistent hypotension, dopamine was injected at a dose of 5–10 µg/kg/min.
An electrocardiogram (ECG) was performed pre and post-procedurally, at 24 hours and 72 hours, for arrhythmias and new ECG changes. As per institutional protocol, oxygen supplementation was given to all patients undergoing PCI with a simple oxygen mask with oxygen at 4 L/min.
At the end of the study, the on-duty anaesthesiologist gave the filled proforma to the primary investigator. Statistical analysis was performed using Medcalc software. Analysis was completed using analysis of variance (ANOVA) followed by a post-hoc test. Haemodynamic variables and CPK and CPK-MB values were expressed as mean ± standard deviation. A p value <0.05 was considered significant.
RESULTS
Each of the three groups included 80 patients. In D1 there were 48 males and 32 females, in D2 there were 40 males and 40 females, and in C there were 43 males and 37 females. All patients were aged between 40 and 60 years. An EF between 40% and 50% was observed in 67, 60, and 61 patients in groups D1, D2, and C, respectively; an EF between 51% and 60% was observed in 13, 20, and 19 patients in groups D1, D2, and C, respectively.
HR decreased significantly, by around 15%, after the loading dose of dexmedetomidine 1 µg/kg infusion was administered in D1 (p=0.02). HR decreased by approximately 19% after the loading dose of dexmedetomidine 2 µg/kg infusion was administered in D2 (p<0.01) (Table 2). There was a statistically significant decrease in MAP by around 12% after the dexmedetomidine 1 µg/kg loading dose was administered. The decrease was greater, approximately 15%, after the administration of the 2 µg/kg dexmedetomidine loading dose (Table 2).
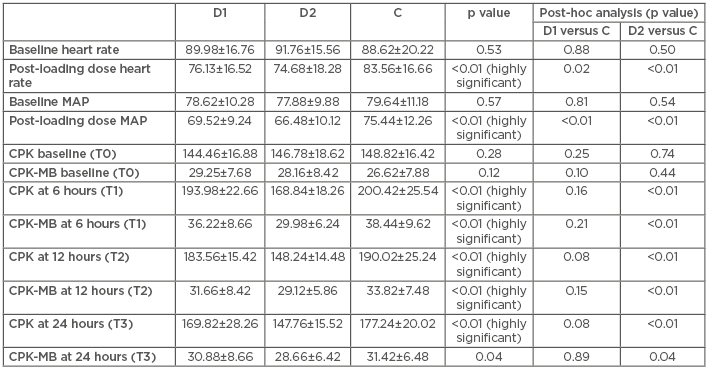
Table 2: Comparison of haemodynamics (heart rate and mean blood pressure), creatine phosphokinase, and creatine phosphokinase-muscle/brain in three groups at four time intervals.
C: control; CPK: creatine phosphokinase; CPK-MB: creatine phosphokinase-muscle/brain; D1: dexmedetomidine 1 µg/kg; D2: dexmedetomidine 2 µg/kg; MAP: mean blood pressure; T: timepoint.
While none of the patients in D1 had a decrease in MAP by <20% and a HR <50 bpm, three patients in D2 had a clinically significant decrease in MAP and five patients had HR <50 bpm, requiring administration of rescue drugs (mephentermine and atropine, respectively).
There was no statistically significant difference in the CPK and CPK-MB values at T0, T1, T2, and T3 between the D1 and C groups (Table 2). However, there was a statistically significant difference in the CPK and CPK-MB values at T1, T2, and T3 between the D2 and C groups (Table 2). Five patients in C had arrhythmias during and post-procedure. In comparison, two D1 patients had arrhythmias and none were reported for D2 patients. All patients in D1 had a modified RSS of either 3 or 4, while patients in D2 had a modified RSS of either 4 or 5. No patient in either group had a reduction in blood oxygen saturation.
DISCUSSION
Dexmedetomidine has been proven to have various advantages in addition to its sedative and analgesic properties. Dexmedetomidine has anti-inflammatory effects when used as an adjunct to general anaesthesia and leads to a significant reduction in serum IL-6, IL-8, and tumour necrosis factor-α levels within a 24- hour period postoperatively.24 Dexmedetomidine protects cardiomyocytes against hypoxia/ reoxygenation injury by suppressing TLR4-MyD88-NFκB signalling.25 Studies have shown that dexmedetomidine protects against oxygen-glucose deprivation/reoxygenation injury-induced apoptosis via the p38 MAPK/ERK signalling pathway.26 However, few studies have failed to demonstrate the myocardial protective effect of dexmedetomidine in the usual recommended doses as far as cardiac enzymes are concerned.22,27
The authors earlier postulated that dexmedetomidine 1 µg/kg loading dose may have a beneficial effect by maintaining the supply:demand ratio, although no beneficial effect was seen on the cardiac enzymes. Dexmedetomidine has been shown to decrease the coronary vessel diameter, at the same time it decreased the heart rate, thus maintaining a balance in the supply and demand of oxygen.22
In the present study, it was observed that a 2 µg/kg dexmedetomidine loading dose has a myocardial protective effect when compared to a 1 µg/kg dexmedetomidine loading dose, with regard to myocardial enzymes. This myocardial protective effect comes at a cost of a greater reduction in HR and MAP, along with a higher sedation score seen in the D2 patients.
Many studies regarding the myocardial protective effect of dexmedetomidine have been carried out in animal models. In one such study in anaesthetised pigs by Yoshitomi et al.,28 it was shown that a high dose of dexmedetomidine (100 ng/mL of coronary blood flow) had a favourable subendocardial:subepicardial blood flow ratio, resulting in better functional recovery from myocardial stunning. In a rat study by Okada et al.,29 dexmedetomidine was shown to have a direct dose-dependent cardioprotective effect on reperfusion injury. Dexmedetomidine improved the infarct size and exhibited a cardioprotective effect on global ischaemia; thus, it was thought that a dose higher than the routinely used 1 µg/kg loading dose is required to elicit a myocardial protective effect in patients undergoing PCI.
Chi et al.21 observed that a 1 µg/kg dexmedetomidine dose reduced myocardial damage in patients undergoing off-pump coronary artery bypass graft surgery, as noted by a decrease in the myocardial enzymes in the dexmedetomidine group when compared to the control group. Analysis was carried out to uncover the possible reasons as to why the same dose of dexmedetomidine could not replicate the results in PCI patients. Firstly, the subgroup of patients in this study were conscious, coming from the ward or intensive care unit with different anxiety levels compared to the anaesthetised surgical patients. Secondly, the dosage required in PCI patients may be different because of the shorter duration of the procedure; the total duration of the procedure was less than the time for normal cardiac surgery. The total dosage of dexmedetomidine infused into the patient was, therefore, reduced in PCI patients compared to the cardiac surgical patients, even though the same maintenance dosing regime was used. Thirdly, the sample size was calculated based on previous surgical studies: a larger sample size may be required in PCI patients. Further study with a different dexmedetomidine dose and with a larger sample size is required to validate the myocardial protective effect in ischaemia reperfusion injury.
Although a myocardial protective effect was elicited by a loading dose of 2 µg/kg dexmedetomidine, it was also associated with a greater number of side effects. In the present study, HR decreased by 15% in D1 and decreased by 19% in D2. Similarly, MAP decreased by 12% in D1 but decreased by 15% in D2. None of the patients in D1 had a decline in MAP <20% and HR <50 bpm, but three D2 patients had a clinically significant decrease in MAP, and five patients had a HR <50 bpm, requiring administration of rescue drugs (mephentermine and atropine, respectively).
Dexmedetomidine caused conscious sedation from which the patient could be woken up easily when stimulated in D1. However, the patients in D2 were more sedated than in D1, warranting greater care in the recovery room. Thus, it is beneficial to use dexmedetomidine 2 µg/kg for myocardial protection in PCI patients, but the beneficial effects come at the cost of requiring greater vigilance by the nursing staff during and post-procedure.
In the present study, five control patients had arrhythmias during or post-procedure compared to two in D1 and none in the D2. There is a probability that dexmedetomidine could have prevented the arrhythmias caused by ischaemia/reperfusion in the D1 and D2 patients in a dose-dependent manner, but the sample size may not be adequate to conclusively prove that.
One of the limitations of the present study was that troponin was not used as a marker of myocardial ischaemia-reperfusion injury. This was because troponin is a paid investigation in the present study institute. In view of the large sample size and the paucity of funds, it was decided to use another marker of myocardial injury, i.e., CPK-MB, which has also been used in previous studies.20,21,23 Another limitation was that long-term patient follow-up was not done in the present study.
CONCLUSION
Dexmedetomidine 2 µg/kg provides a myocardial protective effect as compared to 1 µg/kg, but at the cost of a greater fall in HR and MAP and with a higher sedation score, warranting careful monitoring in the post- procedure recovery room. Large-scale clinical outcome trials are needed before recommending use of a higher dose of dexmedetomidine to confirm its safety and myocardial protective efficacy.