Abstract
Many autoimmune diseases, such as lupus and Sjögren’s syndrome, have a female bias and adult onset. One possible explanation for this bias is disruption of the inactive X chromosome, which is a major epigenetic feature in female cells. Indeed, only one X chromosome is needed in male and female somatic cells because most X-linked genes are not sex-specific. Therefore, one of the two X chromosomes in each female cell is inactivated and appears as a heterochromatic body near the nuclear membrane. It has also been reported that the inactive X is often in close association with a nucleolus, as if nucleoli help maintain the inactive state. The main function of nucleoli is to assemble ribonucleoprotein complexes (RNPs) such as ribosomal subunits and splicing components. For that purpose, nucleoli have high levels of polyamines which assist with the folding and assembly of RNPs. However, as observed under abnormal circumstances such as cellular stress, the nucleolus is very active and can expand dramatically, potentially engulfing the inactive X, which is sandwiched between the nuclear membrane and the nucleolus. As a consequence, polyamines present in the nucleolus could stabilise autoantigenic complexes including those arising from disruption of the inactive X, or autosomes that contain nucleolar organising regions that keep those chromosomes near nucleoli. This suggests that a variety of seemingly unrelated autoantigens can occur in autoimmune diseases through this scenario. In fact, many autoantigens are, at least transiently, components of the nucleolus. Here, with particular emphasis on the inactive X chromosome, we discuss the ‘nucleolus’ hypothesis in which disruption of chromatin due to abnormal nucleolar exposure can lead to autoimmune diseases.
INTRODUCTION
More than 80 autoimmune diseases have been identified, affecting 5–8% of the population as a whole, but many of these diseases share common triggers, symptoms, and patterns making differential diagnosis difficult.1 For example, both systemic lupus erythematosus (SLE) and rheumatoid arthritis (RA) exhibit autoantibodies targeting histones and Z-DNA, which is an infrequent conformation compared with B-DNA.2-4 As another example, an increase in citrullinated proteins occurs in RA (citrullinated collagen) and multiple sclerosis (MS) (citrullinated myelin basic protein), suggesting possible common abnormalities in the functioning of peptidylarginine deiminase enzymes which convert arginine residues to citrulline in special circumstances such as NETosis.5,6 These common aspects of autoimmune diseases can provide hints about their underlying mechanisms.7 The fact that the majority of patients with SLE, RA, MS, and Sjögren’s syndrome (SjS) are female suggests involvement of the X chromosome (Table 1). The fact that SjS can be a primary disease or secondary to SLE, RA, or MS, suggests commonalities among these four diseases.8 Another common aspect is that they have adult onset and episodes can be triggered by stressful events, such as infections.9,10 Adult onset suggests manifestation may arise from accumulation of damage by repeated stresses to cells of the immune system (immune-driven) or cells targeted by the immune system (antigen-driven). The diseases could have a similar mechanism at the cellular level, but differ in symptoms due to occurrence in different tissues or sites with more or less access to the immune response and to systemic circulation of autoantigen-autoantibody complexes, for example, behind the blood-brain barrier versus circulating in the blood. Although a genetic basis is suspected and some statistically significant genetic associations have been reported, in most cases the specific mutations considered to be the main causes of autoimmune diseases have not been found; this suggests that genetic differences may be contributing factors.11,12 As a result, the scope of hypotheses and research have recently expanded to include possible epigenetic involvement, because epigenetic changes can accumulate under environmental influence and lead to altered gene expression without there necessarily being associated genetic mutations.13-19
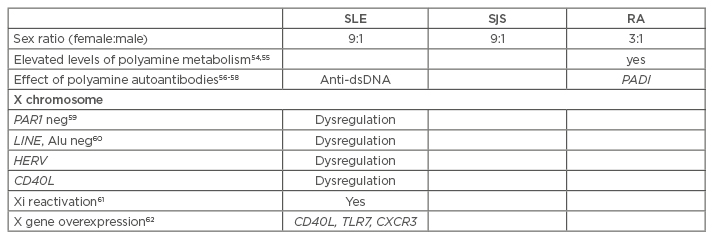
Table 1: Arguments supporting the nucleolus hypothesis with regards to the inactive X chromosome in autoimmune diseases.
SLE: systemic lupus erythematosus; SjS: Sjögren’s syndrome; RA: rheumatoid arthritis; HERV: human endogenous retroviruses; Anti-dsDNA: anti-double stranded DNA; TLR7: toll-like receptor 7; CXCR3: C-X-C motif chemokine receptor 3; PADI: peptidylarginine deiminase.
THE INACTIVE X CHROMOSOME
Most genes on the X chromosome are not sex- specific. Therefore, female cells with two (or more) X chromosomes only need one active X to give the same expression levels of X-linked genes as seen from the one X in male cells. Attaining this equivalency of X-linked expression is referred to as X-linked dosage compensation. Early in embryogenesis, each female cell randomly chooses to inactivate either the paternally-derived or the maternally-derived X. Then each daughter cell will inherit this choice so that the adult is a mosaic of areas where all cells have the paternally-derived X inactivated and, in a neighbouring area, all cells will have the maternally-derived X inactivated. Expression and accumulation on the inactive X of the X-inactive specific-transcript RNA is a major step in establishing X inactivation;20 it recruits epigenetic silencing factors such as histone deacetylases and DNA methyltransferases to the inactive X. The end result is that the inactive X is a dense heterochromatic structure with 75–85% of its genes epigenetically silenced.21,22 The inactive X localises to the nuclear periphery against the nuclear membrane, as if it has been pushed aside by more active chromosomes. This puts the inactive X in a vulnerable situation as the periphery is less accessible for DNA repair, replication, and methylation. The inactive X is the last chromosome to complete replication in late S phase, but requires more effort for DNA repair and methylation, scaffold attachment, and repackaging at a time when the methyl donor, S-adenosylmethionine (SAM), may be at low levels due to recent methylation of other chromosomes.
Adding to these difficulties, the X has fragile sites that can contain chromosome breakpoints or unresolved alternate DNA conformations that impede progression of repair and replication. Completion of replication can be delayed in some regions of the inactive X until late S phase or early Gap 2.23 This can lead to loss of genes (from chromosome breaks), unequal distribution of chromatin to daughter cells, or reactivation of previously silenced DNA when epigenetic control cannot be properly re-established. With age and repeated episodes of stress, there can be chromatin opening and loss of dosage compensation, i.e. gene reactivation on the inactive X.
THE NUCLEOLUS AND POLYAMINES
The nucleolus is a prominent nuclear component, appearing in stained cells as an area mostly devoid of DNA in the nucleus, but the nucleolus does not have a membrane to act as a strict selective barrier. The nucleolus is one of the most active, dynamic, and multifunctional cellular structures, frequently changing its contents and size depending on the cell’s demands for RNA processing and protein synthesis.24 The main function of the nucleolus is to fold RNA transcripts and assemble ribonucleoprotein complexes (RNPs), such as ribosomal subunits, splicing components, transfer RNA (tRNA), signal recognition particles, and small nuclear RNAs.25,26 Some RNA transcripts are expressed in the nucleolus while others are transported into the nucleolus. Folding and macromolecular complex assembly that occurs in the nucleolus requires sufficient open space free of most chromatin, so that large linear RNA transcripts can be folded properly and bind specific proteins required in the RNP.
The Sicca syndrome (SS)-A/Ro and SS-B/La antigen proteins are found in the nucleolus where they act as chaperones that assist in movement and maturation of RNA transcripts and assist in identifying and refolding misfolded transcripts. Nucleolin is a multifunctional protein comprising 10% of the nucleolar proteins. Among its functions, nucleolin assists in chromatin decondensation and assists in expression and assembly of ribosomal subunits. SS-A/Ro, SS-B/La, and nucleolin are frequently targeted as autoantigens in SLE, SjS, and scleroderma.27-29
The nucleolus also contains high levels of the polyamines spermidine and spermine compared to the rest of the cell (Figure 1A, top), which assist in RNA folding in the nucleolus. Polyamines are suspected of having a role in autoimmune diseases.30 RNA transcripts in the nucleolus have an abundance of negatively charged phosphate groups along their length such that self-repulsion makes the RNA incapable of tight folding on its own. Therefore, there is a need for polyamines as counter ions to assist in the folding. Polyamines have unique combinations of length, flexibility, and high positive charge that are beneficial in many cellular functions.31,32 This allows a polyamine to bind and neutralise several neighbouring phosphates in RNA to facilitate bending and intrastrand hybridisation of bases to form helices. These polyamine and RNA interactions are usually transient but folding can persist long enough for further folding or protein binding to occur. In some cases polyamines remain bound in the final tertiary structure, such as spermine bound in the bend of tRNA between the acceptor stem and the anti-codon arm.33,34 The importance of polyamines in folding and assembly in the nucleolus is supported by the facts that polyamines are at their highest levels in the nucleolus and dramatic increases in the size of nucleoli during cell growth and stress response correspond directly to increased polyamine synthesis.35-37 Cells need to synthesise proteins and RNPs that will help in recovery from stress or support growth, thus there is increased demand for nucleolar activity requiring polyamines. Viruses also want to take over a host cell’s nucleoli to produce viral components which require nucleolar products. Therefore, active viruses induce increased polyamine synthesis as an initial step in taking over control of the nucleoli.
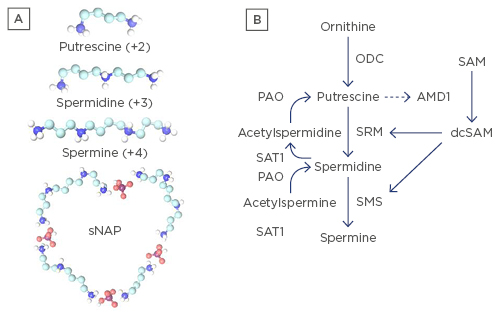
Figure 1: Polyamines.
A) Polyamines: spermidine (+3), spermine (+4), the precursor putrescine (+2), and depiction of a small nuclear aggregate of polyamines (sNAP) containing phosphate ions (believed to be HPO4-2), putrescine, spermidine and spermine. NAPs have been observed in vitro and in vivo;39 B) Polyamine synthesis and recycling pathways. Ornithine is converted to putrescine by ornithine decarboxylase (ODC). Putrescine can allosterically increase S-adenosylmethionine decarboxylase (AMD1) activity to convert S-adenosylmethionine (SAM) to decarboxylated SAM (dcSAM) competing with cellular methylation for SAM. dcSAM provides aminopropyl groups for polyamine synthesis by SRM (spermidine synthase) and SMS (spermine synthase). Polyamines can be recycled with acetylation by SAT1 (spermidine/spermine N1 acetyltransferase) followed by oxidation by polyamine oxidase (PAO). Therefore, two routes exist for generating putrescine, which is normally at only trace amounts to control polyamines. ODC is one of the most tightly controlled enzymes in cells to control polyamine synthesis. Note: SMS (synthesis) and SAT1 (recycling) genes are at Xp22.1 and are normally inactive on the inactive X.21 Reactivation of SMS or SAT1 from the inactive X could lead to detrimental consequences, such as formation of NAPs.
Although polyamines are essential in many functions, polyamine synthesis and recycling must be tightly controlled to avoid potential detrimental effects (Figure 1B). Putrescine, the precursor of spermidine, is normally kept only in trace amounts in the cell to suppress polyamine synthesis as putrescine can bind an allosteric site in S-adenosylmethionine decarboxylase (AMD1) increasing AMD1 activity.38 AMD1 converts SAM to decarboxylated S-adenosylmethionine (dcSAM). SAM is the cellular methyl donor whereas dcSAM cannot be used as a methyl donor. Polyamine synthesis therefore competes directly with cellular methylation, which is important in epigenetic control of gene expression. Overexpression of polyamines could potentially lower SAM levels sufficiently such that some epigenetic silencing could be lost, allowing reactivation of genes with unpredictable consequences. Putrescine must also be kept low to avoid formation of nuclear aggregates of polyamines (NAPs) (Figure 1A, bottom). NAPs are complexes of phosphate ions with putrescine, spermidine, and spermine. NAPs have been observed in vivo and are suspected of binding cellular macromolecular complexes, possibly stabilising them in autoantigenic forms which may be more protected from nucleases and proteases by NAPs compared with individual polyamines (Figure 2).39
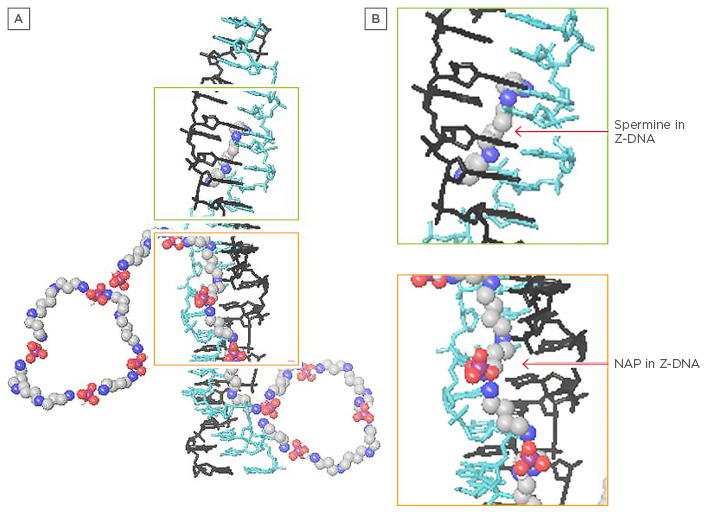
Figure 2: Z-DNA with spermine and nuclear aggregates of polyamines.
A) Depiction of Z-DNA with spermine and NAPs; B) Close-up. Note how NAPs could bind in the Z-DNA minor groove with polyamines, assisting each other and providing more resistance to nucleases and stability to potentially autoantigenic conformations.
NAPs: nuclear aggregates of polyamines.
Two polyamine genes, spermine synthase (SMS) and spermidine/spermine N1 acetyltransferase (SAT1), are located on the X chromosome at Xp22.1. Normally SMS and SAT1 are silenced on the inactive X.21,40 However, loss of dosage compensation could lead to SMS and SAT1 overexpression. This could lead to wasteful synthesis and recycling of polyamines, wasting SAM needed for methylation. SAT1 can undergo superinduction, (i.e. expression increased several hundred fold).41 In polyamine recycling, SAT1 acetylates spermine to acetylated spermine, which is oxidised to spermidine, and spermidine can be acetylated and oxidised to putrescine, thereby providing an abnormal increase in putrescine that can allosterically increase AMD1 activity to support new polyamine synthesis. This alternate route of putrescine production via polyamine recycling could support NAP formation. In addition, further oxidation of putrescine can create acrolein, a very toxic compound, the appearance of which shows a strong correlation with the intensity of SjS.42 Therefore, maintenance of the inactive X is important to avoid consequences of abnormal polyamine synthesis and recycling. Increased polyamines and acetylated polyamines could throw off efficient nucleolar functioning and cause misfolding, misassembly, and stabilisation of abnormal conformations of DNA, RNA transcripts, and associated proteins. Persistence of these structures in potentially autoantigenic forms could lead to an autoimmune response. For example, the SS-A/Ro and SS-B/La proteins and nucleolin chaperone RNA transcripts and associated proteins through nucleolar processing. These proteins also help identify abnormal folding and assembly. Recently, the SS-B/La autoantigen has been demonstrated to be dysregulated at the epigenetic level in salivary gland epithelial cells from patients with primary SjS.43-45 As such, they could be frequent common epitopes within larger complexes that contain autoantigenic epitopes. SS-A/Ro, SS-B/La and nucleolin could be targeted because of ‘guilt by association’ with misfolded RNAs stabilised by an overabundance of polyamines.
THE ‘NUCLEOLUS’ HYPOTHESIS
The inactive X has been reported to be in close association with nucleoli in 90% of cells in S phase and about one-third of cells in the overall cell cycle, except for mitosis during which the nucleoli disappear.46 Expansion of the nucleolus due to cellular stress could potentially involve engulfment of some or all of the inactive X (Figure 3).34 This would expose the inactive X to high levels of polyamines in the nucleolus, and there could be putrescine and NAPs from abnormal polyamine synthesis and recycling. Some of the inactive X is euchromatic, such as the PAR1 region that escapes inactivation.47 Inactive peptidylarginine deiminase 4 (PAD4), a calcium-activated enzyme that converts arginine residues into neutral citrulline, localises to euchromatin. When PAD4 is activated such as during NETosis, citrullinated histones lose their hold on DNA resulting in chromatin opening. We have proposed previously that polyamines could activate PAD4 in place of calcium.48 For example, two calcium binding sites are 13.5 Å apart, which could potentially bind spermine. The other three calcium binding sites are in alignment spanning 11.0 Å which is similar to the length of spermidine. Thus, PAD4 associated with the inactive X could become active when exposed to high nucleolar polyamine levels. This would open more of the inactive X, allowing reactivation of genes. As the nucleolus returns to normal, newly opened X-linked chromatin could bind transcription factors and polymerases. Of particular concern is a high concentration of Alu elements in PAR1. Alu elements comprise ~10% of the human genome but 28.8% the PAR1 region of the X.49 Normally these ≥2,500 Alu elements would be silenced by a positioned nucleosome but exposure to high levels of polyamines and polyamine- activated PAD4 could lead to expression of these Alu elements by RNA polymerase III (Pol III). The SS-A/Ro and SS-B/La proteins associate with Pol III transcripts to assist maturation, including the refolding of misfolded transcripts. This sudden appearance of Alu transcripts could take up the available SS-A/Ro and SS-B/La, hampering maturation and refolding of other Pol III transcripts. In addition, Alu transcripts can form intrastrand hybridisation that can be stabilised by polyamines in potentially autoantigenic forms or they can compete with the Alu domain of the signal recognition particles for proteins.34 When released, negative supercoiling stress previously stored in nucleosomes on the Alu elements would flux through the DNA and potentially form Z-DNA that could be stabilised by polyamines and NAPs. In addition, there is a fully functional LINE1 reverse transcriptase in the inactive X short arm.34 If this is expressed, it could reverse transcribe Alu transcripts to create hypomethylated Alu DNA. Li and Steinman50 reported a high percentage of Alu DNA in the free DNA of lupus patients’ sera and proposed that it could be reverse-transcribed DNA.
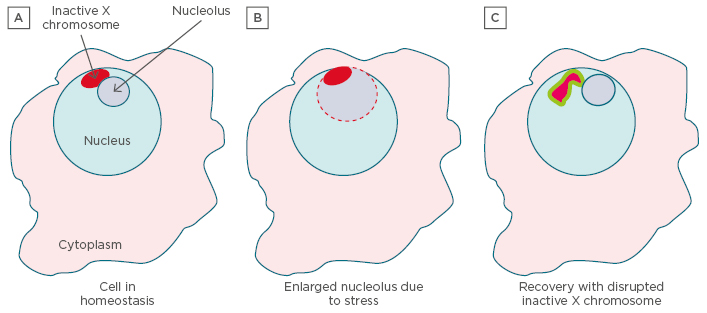
Figure 3: The ‘nucleolus’ hypothesis.
A) The inactive X (red) is in close association with a nucleolus (grey) in 90% of cells in S phase and one-third of cells throughout the cell cycle.46 This puts one of the most inactive components of the nucleus, the inactive X, next to one of the most active and dynamic components, the nucleolus. Normally the inactive X is located at the nuclear membrane. B) Stress can cause a rapid increase in the size of the nucleolus as it is called on to produce more ribonucleoproteins needed for recovery. Increased nucleolar size is directly related to increased polyamines which assist in folding and assembly of ribonucleoproteins in the nucleolus.35 The enlarged nucleolus could engulf the inactive X, exposing it to high levels of polyamines and NAPs. Inactive PAD4 localised in euchromatin (such as PAR1 of the inactive X47) could be abnormally activated by binding polyamines instead of calcium.48 PAD4 could open previously sequestered genes. C) With cell recovery, X-linked genes may be overexpressed including reactivated genes on the inactive X (green border). Abnormally high levels of polyamines and NAPs, along with reduced cellular methylation, could lead to generation of abnormal DNA, RNA, and RNP conformations stabilised by polyamines and NAPs in autoantigenic forms.
NAPs: nuclear aggregates of polyamines; PAD4: peptidylarginine deiminase 4; RNP:
ribonucleoprotein complex.
IMPLICATIONS
This discussion has focussed on the inactive X and nucleolus association, which could explain female predominance of some autoimmune diseases, yet we should keep in mind that chromosomes 13, 14, 15, 21, and 22 have nucleolar organising regions that keep them in close association with the nucleolus. They could also be disrupted by a nucleolar stress response and result in similar consequences as described for the inactive X chromosome.
Alu elements and other repetitive elements such as human endogenous retroviruses are important in the hypothesis,51-53 particularly the high Alu concentration in PAR1 of the X. Mice do not have any significant amounts of Alu elements; the mouse X consists of only a long arm and no short arm, so inactivation is established relatively consistently on the mouse X. As such, it has been difficult to study partial X reactivation in mice. The human X has both a short and long arm separated by a centromere, making the X inactivation state less consistent. These differences between human and mouse X chromosomes explain why studies on X-linked epigenetics in autoimmune diseases are problematic when using mouse models.
Finally, the close proximity of a functional LINE1 reverse-transcriptase to a high concentration of Alu elements in the X short arm has very interesting possibilities with regards to retrotransposition events and how stress could trigger such events.
CONCLUSIONS
The ‘nucleolus’ hypothesis provides a comprehensive explanation for: i) the female bias of many autoimmune diseases, ii) generation of a broad range of autoantigens many of which are at least transiently in the nucleolus, iii) a common mechanism that can relate to different autoimmune diseases, and iv) major involvement of epigenetics in autoimmune diseases.