Abstract
Type 2 inflammation is involved in severe asthma (SA), chronic rhinosinusitis with nasal polyps (CRSwNP), and eosinophilic granulomatosis with polyangiitis (EGPA). The pathogenesis of these diseases involves various immune system cells and target tissues, along with numerous cytokines, chemokines, and other inflammatory molecules. The role of eosinophils and the cytokine IL-5 and its receptor (IL-5R) is becoming a significant focus in these diseases, with numerous studies, from in vitro experiments to clinical trials, demonstrating correlations between IL-5 levels with eosinophil numbers and activity in SA, CRSwNP, and EGPA. Eosinophils and IL-5 are involved, both directly and indirectly, in disease initiation and exacerbation, as well as in tissue remodelling and disease progression. An advisory board of experts discussed how understanding the actions of IL-5 within Type 2 inflammation in SA, CRSwNP, and EGPA can highlight the importance of its role in managing patients with these diseases and help identify suitable biomarkers for disease onset, exacerbation, and progression. Therapies targeting eosinophil generation, priming, and activation could lead to more effective disease control and help prevent the tissue damage associated with these conditions, which can be difficult to reverse in chronic cases.
INTRODUCTION
Type 2 immunity involves both adaptive and innate immune responses to pathogens and toxic substances. However, when these mechanisms are upregulated in response to an innocuous allergen or stimulus, a range of Type 2-associated inflammatory diseases can occur. Type 2 inflammation plays a role in triggering and progression of SA, CRSwNP, and EGPA. These diseases affect airway epithelium and show some common features including inflammatory cell trafficking into airway tissues, epithelial barrier disruption, goblet cell hyperplasia, increased mucus production, and tissue remodelling (Figure 1).1,2
One mechanism behind Type 2 inflammation is characterised by the production of IgE by B cells, following their activation by T-helper 2 (Th2) cells (Figure 1).1,2 This mechanism also involves a number of other immune cells and factors that together contribute to development and progression of SA, CRSwNP, and EGPA. Here though, the authors focus on the cytokine IL-5, whose role in such conditions is highlighted by the efficacy of IL-5-targeting therapies.1 While IL-5 is produced by, and has actions on, a number of immune cells, including Th2 cells, mast cells, and Type 2 innate lymphoid cells (ILC2), another focus here will also be on eosinophils (Figure 1).2 This was prompted by a discussion by a board of experts, who highlighted the importance of understanding the impact of IL-5 and eosinophils in SA, CRSwNP, and EGPA when managing patients with these diseases.3
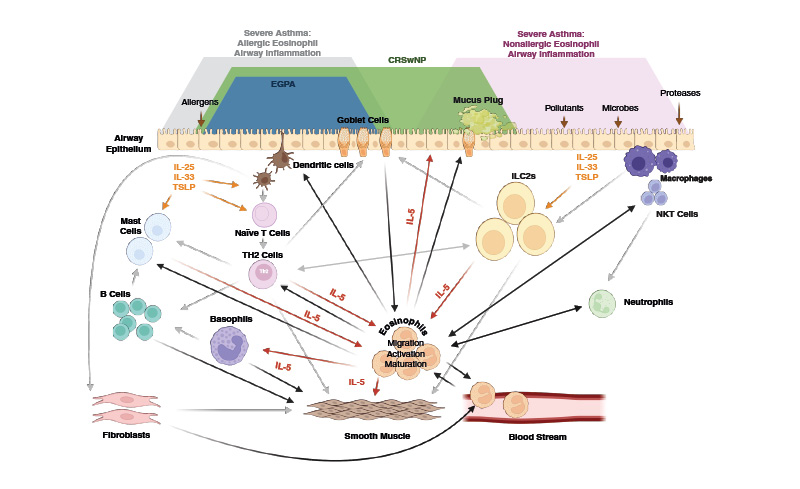
Figure 1: The overarching role of IL-5 and eosinophils in severe asthma, chronic rhinosinusitis with nasal polyps, and eosinophilic granulomatosis with polyangiitis.
CRSwNP: chronic rhinosinusitis with nasal polyps; EGPA: eosinophilic granulomatosis with polyangiitis; IL: interleukin; ILC2s: type 2 innate lymphoid cells; NKT: natural killer T; TH2: T-helper 2; TSLP: thymic stromal lymphopoietin.
EOSINOPHILS AND IL-5 INVOLVEMENT IN TYPE 2 INFLAMMATION
Eosinophils are bone marrow-derived leucocytes found in abundance in SA, CRSwNP, and EGPA, both locally, including in airway epithelium, and remotely (Figure 2).4-6 Even in people without asthma or those who do not smoke, higher blood eosinophil counts (>400 cells/μL) are frequently, although not necessarily, associated with airflow obstruction and lung function decline.7
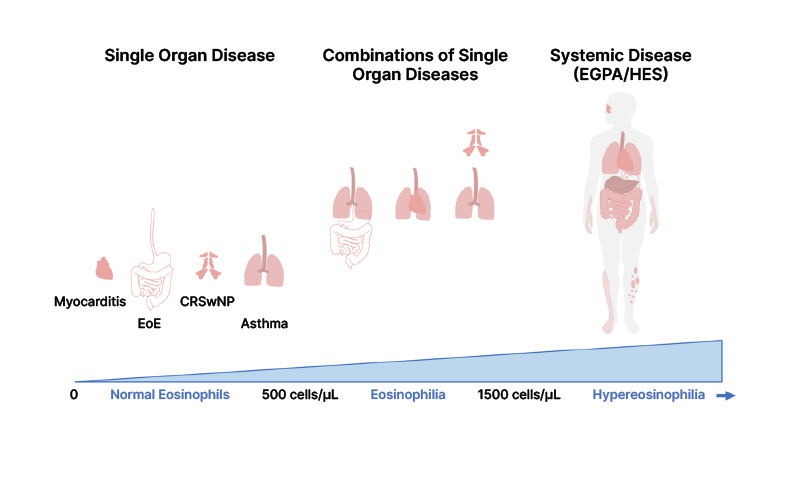
Figure 2: Eosinophil-related disorders according to blood eosinophilia.
Adapted from Lombardi et al.3 2024 under CC-BY-NC-4.0.
CRSwNP: chronic rhinosinusitis with nasal polyps; EGPA: eosinophilic granulomatosis with polyangiitis; EoE: eosinophilic oesophagitis; HES: hypereosinophilic syndrome.
Eosinophils are major releasers of inflammatory mediators, including IL-5 and other cytokines, chemokines, granule proteins, and lipid mediators (Figure 1).8 The presence of IL-5, along with IL-3 and granulocyte-macrophage colony-stimulating factor (GM-CSF), may also enhance the responsiveness of eosinophils to other stimuli and activation of downstream pathways. This can lead to a ‘hyperresponsive’ phenotype including increased levels of eosinophil degranulation and inflammation.9 As part of this, IL-5 expression can upregulate eosinophil extracellular trap-forming cell death, increasing inflammation and tissue damage.10 In this process, released extrudate includes the granule proteins eosinophil-derived neurotoxin (EDN), eosinophil peroxidase, major basic protein (MBP), and eosinophil cationic protein (ECP). Extrudate can also include chromatic structures (‘DNA traps’ or ‘eosinophil extracellular traps’), cytokines (including IL-5), chemokines, enzymes, and growth factors (Figure 1).8,11
Eosinophils have numerous downstream and bidirectional effects that contribute to the pathogenesis of Type 2 inflammation.8 For example, they can act together with mast cells in allergic reactions, with histamine released from such cells inducing eosinophil chemotaxis (Figure 1),12 and can stimulate lymph node T cells to produce IL-5 following an allergen challenge.13 Eosinophils may also have a direct role on both naïve and memory B cell proliferation, secretion of IgA, IgG, and IgM from B cells, and B cell survival, in peripheral blood and tissues. In vitro studies show that this may occur through direct contact or, to a lesser extent, through contact of B cells with eosinophil-derived soluble factors, independently from other B cell modulators such as T cell derived cytokines.14
Eosinophil activity is primarily directed by IL-5 through the IL-5Ra subunit. This is specific to IL-5, unlike the beta-subunit, which is also shared by IL-3 and GM-CSF.15 IL-5 and IL-5Ra play a significant role in eosinophil growth, maturation, and differentiation in the bone marrow; in eosinophil proliferation, activation, and recruitment throughout the body; and eosinophil survival and mortality.15-17 The IL-5R is also expressed, to a lesser extent, on other immune cells, such as neutrophils, basophils, B cells, and mast cells, as well as on non-immune cells associated with the airway epithelium, including ciliated epithelial cells and lung fibroblasts (Figure 3).16,18-21 This widespread activity is postulated to underpin the sometimes broad effects of IL-5 targeting treatments.1
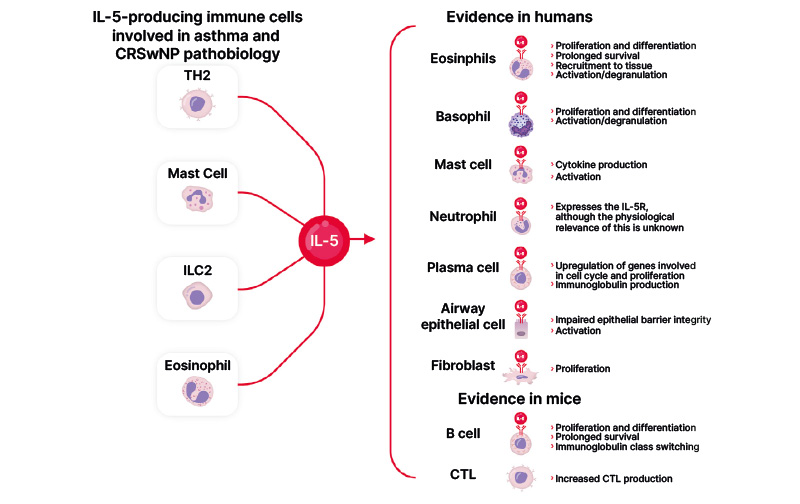
Figure 3: IL-5 signalling in different cell types.
Adapted from Bachert et al.2 2023
CRSwNP: chronic rhinosinusitis with nasal polyps; CTL: cytotoxic T lymphocyte; IL: interleukin; ILC2: innate lymphoid cell type 2; TH2: T-helper 2 cells.
SEVERE ASTHMA AND CHRONIC RHINOSINUSITIS WITH NASAL POLYPS
CRSwNP and SA are respectively characterised as upper and lower airway diseases. Disruption to the respiratory system can occur due to various inducers, including allergens, and triggers, such as smoking, infections, and pollution. When such inducers and/or triggers are present at the airway epithelium, the ‘alarmins’ IL-25, IL-33, and thymic stromal lymphopoietin signal to eosinophils, ILC2s, dendritic cells, and Th2 cells. These can, in turn, secrete Type 2 cytokines, including IL-5 (Figure 1).1,15
IL-5 and Eosinophils in Severe Asthma
Asthma symptoms can range from mild, episodic wheezing to chronic airway narrowing and life-threatening episodes. These symptoms are caused by the actions of innate and adaptive immune cells, along with epithelial cells, causing airway hyperresponsiveness, inflammation, excessive mucus production, and tissue remodelling.22 Of the approximately 300 million people worldwide with asthma,22 an estimated 5−12% have a chronic, severe form (SA), which in some may be uncontrolled.23 Despite advances in management over the decades, there are globally an estimated 1,000 deaths a day due to asthma.22
At least 84% of adults with SA may have eosinophilic ‘T2-high’ asthma,5 characterised by blood eosinophils ≥150 cells/μL, the presence of tissue eosinophils, IgE >114 kUL-1, and fractional concentration of exhaled nitric oxide (FeNO) >19.5 parts per billion (though of note, precise levels of each may differ between guidelines).1 People with eosinophilic SA are more likely to be female, have adult-onset asthma and nasal polyps,5 and have higher levels of blood and sputum eosinophil progenitors, ILC2s, and CD4+ T cells.24
Eosinophilic inflammation in people with asthma is associated with worse lung function and airflow obstruction over time compared to those without such inflammation.25 In vitro studies show that eosinophils from people with asthma can induce smooth muscle cell contraction, potentially contributing to asthma symptoms.26 Typically, people with eosinophilic asthma require high doses of oral steroids to achieve disease control and they may be refractory to inhaled corticosteroids.22 Notably, there is an approximately 1.4-fold increase in the likelihood of ≥2 exacerbations if blood eosinophil count is >400 cells/μL, compared with ≤400 cells/μL, suggesting that levels of these cells could be used to predict asthma exacerbation risk.27 This risk can more comprehensively be calculated by also taking into account FeNO and presence of clinical risks, such as comorbidities or environmental exposure.28
Two distinct subtypes of eosinophils have been identified in vivo with regard to airway diseases: IL-5-dependent inflammatory eosinophils (iEOS) and non-IL-5-dependent tissue-resident eosinophils (rEOS).29-31 In eosinophilic SA (or models of such), iEOS are found in peripheral blood, nasal polyps,30 and the lungs.29 In peripheral blood, iEOS have increased activation markers and in nasal polyps they have increased IL-3R and IL-5R, with the percentage of iEOS correlating with eotaxin-3 expression.30 There is significant correlation between peripheral blood iEOS proportion and asthma severity, exacerbations, and nasal polyp numbers.31 In general, in patients with asthma, the proportion of circulating iEOS to total eosinophil count is significantly higher compared with healthy controls.32 Following a trigger, iEOS can infiltrate the airways from the blood.29 However, with IL-5 inhibition and gains in asthma control, there is a reduction of iEOS and an increase in rEOS.31
Granular proteins and DNA traps released from eosinophils can increase airway inflammation in SA33 and damage structural cells in the lung.34 These proteins are involved in airway remodelling by modulating fibroblast and smooth muscle cell activity, leading to muscle hyperplasia and hypertrophy.35 Studies on specific granular proteins have shown that MBP levels are significantly elevated in sputum from patients with asthma,36 especially during exacerbations,36 and that eosinophils and MBP are abundant in bronchial tissue in cases of fatal asthma.37 Further, ECP is raised in serum in allergen-provoked asthma.38 With this in mind, granule protein levels could be useful biomarkers for SA exacerbations.
Mucus plugs are present in many patients with asthma, causing airflow obstruction. They can significantly contribute to SA pathology and to fatalities during asthma exacerbations,39,40 so are a critical therapeutic target in SA. There is a correlation between mucus plug development and eosinophil number, IL-5 expression, and eosinophil peroxidase levels. The latter is associated, alongside reactive oxidants, with changes to mucin polymers shown in mucus plugs, with eosinophil granule proteins as a whole associated with increased mucus viscosity. These elements combined also exhibit decreased degradation to usual mucus-eliminating factors such as proteases, and increased contribution to inflammatory mechanisms.39,41 Another marker of eosinophilic inflammation is the formation of ‘Charcot–Leyden crystals’ (CLC) from galectin-10. Combined, CLCs and DNA traps can also form part of mucus plugs42 (Figure 1) where they contribute to airway obstruction impenetrable to bronchodilators.39
Several findings highlight the role of IL-5 in asthma. For example, this cytokine is upregulated in bronchial mucosa following allergen exposure,43 and serum IL-5 levels are higher in people with SA compared to those with mild or no asthma.44 During asthma exacerbations, IL-5 and eotaxin expression can promote eosinophilic recruitment to airway epithelium, leading to airway hyperresponsiveness.16 Not only during attacks but also in people with stable asthma, IL-5 levels are inversely correlated with apoptotic eosinophil counts.45 In allergic asthma, eosinophil numbers correlate with high levels of IL-5 in sputum, serum, peripheral blood T cell supernatants, and bronchoalveolar lavage fluid (BALF).15,46-48 Increased soluble IL-5Ra is also found in BALF following allergen challenge, indicating eosinophil tissue migration across the epithelium, as the receptor is shed at this point.49 Further, FeNO levels correlate with both airway IL-5 and eosinophils in SA, but not blood eosinophil count, suggesting that assessing systemic versus airway Type 2 inflammation may be more accurate when combining blood eosinophil counts with FeNO measurements.50
Of particular importance are correlations between IL-5 and disease pathogenesis and severity. For example, higher IL-5 expression in BALF is associated with increased asthma symptoms, abnormal airway physiology, and decreased pulmonary function.43,51 IL-5 can also promote eosinophil degranulation, associated with bronchial epithelium injury.16,52 That this cytokine may be involved in decreasing epithelial barrier integrity and tissue damage is evidenced by an in vitro study showing that IL-5 signalling to airway epithelial cells can lead to downregulation of adhesion molecules.20 In vivo, IL-5 expression has also been associated with peri-bronchial smooth muscle layer thickening and peri-bronchial fibrosis in airway remodelling, attributed to eosinophil action.53 One possible downstream mechanism for these findings involves tumour growth factor-β, which is secreted by eosinophils in people with SA54 and can promote myofibroblast transformation, leading to airway remodelling.34 Such remodelling is associated with higher asthma medication use, reduced lung function, and airway hyperresponsiveness.55 These findings are significant because they suggest a role for disease modification through IL-5-targeting, glucocorticoid-sparing means. Indeed, blocking IL-5 can lead to a significant decrease in circulating eosinophil levels56,57 and can significantly reduce levels of lung eosinophils in patients with corticosteroid-resistant asthma.56
While there is an intimate relationship between eosinophils and IL-5, it is not exclusive. Airway remodelling can occur without eosinophilic inflammation,55 suggesting that other IL-5-expressing cells may play a role in SA pathogenesis. For example, ILC2 numbers are increased in the airways following an allergen challenge,58 and these cells are a source of IL-5 in the lungs of people with asthma (Figure 1 and Figure 3).59 IL-5 can also enhance histamine release from basophils in vitro;60 however, there is an inverse relationship between rate of activated basophils and effectiveness of IL-5-targeting therapy.61 Further, in asthma, elevated synthesis of airway collagen may be driven by IL-5 activation of fibroblasts and IL-5Ra expression in lower airway lung fibroblasts is significantly greater in people with asthma compared with healthy controls.21 This suggests that cells other than eosinophils could also be a target for decreasing SA manifestations.
IL-5 and Eosinophils in Chronic Rhinosinusitis with Nasal Polyps
Global prevalence estimates for CRSwNP are between 1.1−4.4%.62 Symptoms of CRSwNP include not only nasal polyps but also inflamed mucosa, postnasal drip, frontal sinus pain and pressure, and airway impedance.63 Notably, CRSwNP and asthma may be comorbid,62,64 with comorbidity increasing CRSwNP severity,64,65 asthma exacerbation frequency,64,66 and oral corticosteroid use, and decreasing health-related quality of life.62
CRSwNP predominantly arises due to Type 2 inflammation.67 This may drive nasal polyp formation through oedema, plasma protein retention, and fibrin deposition.68 Several findings point to the role of IL-5 in CRSwNP. Similar to SA, in this disease epithelial cell alarmins can lead to ILC2 activation and to IL-5 accumulation and production (Figure 1).10 Such upregulation of IL-5 in nasal polyps69 correlates with CRSwNP severity.17 Higher levels of IL-5 in nasal secretions and nasal polyp tissue are especially shown in people with uncontrolled CRSwNP, compared to those where it is controlled, with higher nasal secretion of IL-5 correlating with a higher number of polyp surgeries.70
Elevated IL-5 levels have also been shown in nasal polyps associated with aspirin-exacerbated respiratory disease, alongside high numbers of antibody-secreting cells, including plasma cells and plasmablasts. Increased expression of IL5-Ra in such patients is proposed to have a role in local antibody production and is correlated with disease severity and recurrence of nasal polyposis.71,72 That IL-5Ra might have a role in epithelial-mesenchymal cell transition in CRSwNP is evidenced in an investigation of another disease, pulmonary fibrosis, where the receptor was upregulated in such cells as fibrosis progressed.73 Other IL-5R-expressing cells found elevated in people with CRSwNP include IgG+ B cells, basophils, mast cells, and ILC2s, indicating a potential IL-5-linked role of these cells in disease pathology.6
Similar to airway epithelium in SA, the increased presence of eosinophils and other Type 2 inflammatory cells is shown in polyps in CRSwNP.68 Eosinophil-dominant CRSwNP features include dysosmia, nasal discharge, multiple bilateral polyps, involvement of the ethmoid sinus and accompanying asthma, drug allergies, and aspirin intolerance.74 Eosinophilia is more marked in patients where CRSwNP reappears following surgery, compared to a higher neutrophil number in patients where surgery is more successful.74 IL-5 can promote eosinophil-associated inflammation in CRSwNP,75 and upregulation of leukotrienes in eosinophils, as well as in mast cells and basophils, in CRSwNP is potentially via IL-5/IL-5Ra signalling as inhibition of this receptor subunit in patients with CRSwNP results in downregulation of these inflammatory molecules.19,76 Furthermore, the release of IL-5 from eosinophils can lead to B cell Ig class switching.6
The actions of eosinophils in CRSwNP may lead to collagen deposition, fibroblast activation, epithelial damage, and dysosmia.6,77 These may be due to the release of eosinophil granule proteins. For example, in eosinophil-dominant CRSwNP, ECP levels in nasal secretions correlate with polyp severity and the presence of asthma.78 Additionally, EDN is found at high levels in nasal polyps and serum EDN levels are significantly higher in patients with eosinophilic CRSwNP compared with other nasal diseases, correlated with disease activity. IL-5 plays a role here with in vitro stimulation of eosinophils with this cytokine leading to EDN production and stimulation of nasal epithelial cells, and EDN presence resulting in changes in genes associated with epithelial-mesenchymal transition.79 CLCs from eosinophils are also abundant in nasal polyps, co-located in areas of eosinophil extracellular trap-forming cell death, and positively correlating with levels of IL-5.42 Levels of CLC-associated galactin-10 also correlate with CRSwNP severity.80 These findings point to a need to more fully understand the relationship between IL-5 and eosinophils in CRSwNP pathology.
IL-5 AND EOSINOPHILS IN EOSINOPHILIC GRANULOMATOSIS WITH POLYANGIITIS
Also being investigated, though to a lesser extent than has been carried out for SA and CRSwNP, is the role of IL-5 in EGPA, another disease that can have Type 2 inflammatory processes feature in its pathogenesis. Known originally as Churg–Struss syndrome, EGPA is characterised by multisystem tissue eosinophilia, which leads to eosinophil-rich granulomatous infiltration, small-vessel necrotising vasculitis, disease-onset asthma, recurrent rhinitis and sinusitis, and, in some cases, polyposis. Peripheral blood eosinophilia in EGPA can typically be >1,000−1,500 cells/μL, with eosinophil levels correlating with disease activity. EGPA is typically diagnosed in adulthood, with prevalence estimates ranging from 10.7−18.0 per 1,000,000.4,81-84
EGPA can be either antineutrophil cytoplasmic antibody (ANCA)-positive, in around 30−40% of patients who present more often with vasculitic lesions, or ANCA-negative, where eosinophil-related organ infiltration is more prominent.4,81,82 The disease usually progresses through several phases: a prodromal phase dominated by asthma and chronic rhinosinusitis; a phase with increased eosinophil and organ involvement, such as lung infiltrates and cardiomyopathy; and a vasculitic phase, which may include neuropathy, glomerulonephritis, and palpable purpura. These phases may not occur sequentially and can overlap.4,81,82 Immunosuppressive therapies can be used to block vasculitis-associated inflammation, leading to vasculitis remission, but may not impact other phases and/or may lead to treatment-related damage accrual.85
Eosinophils are one of the primary cell types involved in the pathogenesis of EGPA. Increased presence of these cells may lead to tissue damage in EGPA through the secretion of granule proteins.81 For example, eosinophils may have a direct role in EGPA-related axonal neuropathy and prothrombotic endothelial damage, causing irreversible peripheral nerve damage.86 In patients with EGPA-associated cardiac damage, peripheral eosinophilia is also notably pronounced.82 Eosinophils develop from IL-5R-expressing CD34+ progenitor cells, and IL-5-producing CD34+ cells have been found in peripheral blood and bone marrow samples in people with EGPA. As such, the potential benefits of targeting these early-phase cells to reduce the accumulation of tissue eosinophils in EGPA prior to any damage is an unmet need of the disease.87
At least part of EGPA manifestations are mediated by Th2 cells and their associated cytokines, including IL-5, along with IL-4 and IL-13 (Figure 1).4 Understanding IL-5 involvement in EGPA could provide a valuable biomarker for the disease and enhance understanding of disease activity. Studies indicating a role for IL-5 in EGPA include one showing that T cell primed peripheral blood mononuclear cells from patients with EGPA release significantly more IL-5 than cells from healthy controls.88 Another investigation found that increased plasma IL-5 was correlated with EGPA exacerbation and demonstrated that, during active EGPA, IL-5 expression is elevated in BALF compared to people with inactive EGPA and those with asthma, indicating a role of IL-5 in EGPA activity levels. A significant correlation was also found between IL-5 concentration in BALF and both BALF and blood eosinophil levels, indicating the elevated presence of IL-5 in airways during active EGPA.89 A role for B cells in EGPA pathogenesis has also been suggested4 as IL-5 from Th2 cells acting on B cells leads to elevated ANCA, IgE, and IgG4 production in people with EGPA.82 The role of IL-5 in EGPA is further supported by several studies highlighting the effectiveness of IL-5/IL-5Ra-targeting medications for this disease.4,81,82
CONCLUSION
Numerous studies highlight the role of IL-5 and eosinophils in Type 2 inflammation occurring in SA, CRSwNP, and EGPA (Figure 1). Eosinophil activation and number is related to disease severity in these diseases, underscoring the potential benefits of inhibiting eosinophil priming and activation, as well as targeting upstream eosinophil generation.90 Current advances in understanding the complexity of the IL-5 pathway across all relevant cells and targets, not just eosinophils, is beneficial for patient phenotyping and management. Such approaches could lead to more effective disease control, prevent tissue damage and remodelling, help manage chronicity, and improve a patient’s overall health-related quality of life.1,3,6,10,15,21,23,45,52,56,67,68,85,89
NX-IT-IMU-MONO-240001 November 2024