Abstract
Bronchopulmonary dysplasia (BPD) is a chronic inflammatory lung disease that affects thousands of newborns and infants every year. Although it is accepted that BPD results from lung damage and inflammation triggered by mechanical ventilation and hyperoxia, the causes and molecular events leading to lung damage and arrested development remain unknown. While recent advances in neonatal care have improved the survival of very low-weight infants, the rates of BPD have not improved accordingly. This is mainly due to our limited understanding of the disease’s pathogenesis and the effective therapeutic options available. Current therapeutics for BPD involve ventilation management, steroid treatment, and administration of various agents, such as pulmonary surfactant, caffeine, vitamin A, nitric oxide, and stem cells. However, the efficacy of these agents in preventing and ameliorating BPD symptoms varies depending on the populations studied and the disease stage. As the field moves towards personalised therapeutic approaches, this review summarises clinical and experimental studies conducted in various models, aiming to increase understanding of the cellular and molecular mechanisms by which these agents can prevent or treat BPD. Due to the increasing number of extremely premature infants, it is imperative that we continue to work towards understanding the mechanisms of BPD pathogenesis and generating more effective therapeutic options.
INTRODUCTION
Bronchopulmonary dysplasia (BPD) is a chronic inflammatory lung disease of prematurely born infants characterised by impaired lung development and requiring mechanical ventilation with supplemental oxygen. The disease was initially defined by Northway et al.1 in 1967 as a disorder resulting from the combined effects of oxygen exposure and mechanical ventilation in premature infants with severe respiratory distress syndrome. This initial definition was based on time spent on ventilatory support and oxygen concentration, which were thought to induce damage to the lung epithelium, smooth muscle hyperplasia, atelectasis, and vascular hypertension, resulting in an immature and surfactant-deficient lung. As a result of significant improvements in neonatal care, including the use of antenatal steroids, ventilation, and nutritional management, the definition of BPD has been modified in the past few decades to include additional criteria reflecting symptoms of arrested alveolar and vascular lung development, fibrosis, and chronic inflammation.2-4 Despite these changes, some investigators still argue that a definition based on the disease’s pathophysiology should be developed.5 Moreover, recent studies using a variety of biomarkers have provided additional information that could complement current diagnostic strategies and help in the development of a more comprehensive definition.6 The current National Institute of Child Health and Human Development (NICHD) and National Heart, Lung, and Blood Institute (NHLBI) criteria for the BPD definition encompasses gestational age (GA), supplemental oxygen requirement, and chest X-ray changes, categorising BPD by degree of severity.7 A summary of the current diagnostic criteria for BPD severity is presented in Table 1. According to the NICHD and NIH guidelines, BPD is defined as mild, moderate, or severe. In infants with a GA <32 weeks, mild BPD is considered when there is a requirement for at least 28 days of supplemental oxygen, together with termination of supplemental oxygen or discharge by 36 weeks post-menstrual age (PMA). Mild BPD is also considered if termination of ventilation or discharge is achieved by 56 days postnatal age in infants with a GA >32 weeks. In contrast, moderate BPD is considered in infants with a GA ≤32 weeks when there is a requirement of at least 28 days of supplemental ventilation with <30% oxygen at 36 weeks PMA, or by 56 days postnatal age (in infants with a GA ≥32 weeks). In the case of severe BPD, the diagnosis is defined as requiring >30% supplemental oxygen for at least 28 days at 36 weeks PMA or at discharge (for infants with a GA <32 weeks), or at 56 days postnatal age or discharge (for infants with a GA ≥32 weeks).
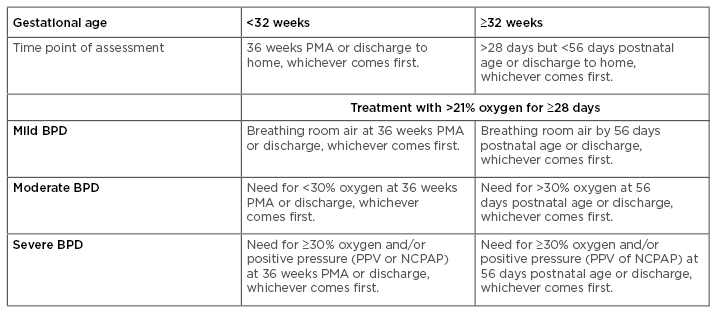
Table 1: Definition of bronchopulmonary dysplasia: Current diagnostic criteria.
BPD: bronchopulmonary dysplasia; NCPAP: nasal continuous positive airway pressure; PMA: post-menstrual age; PPV: positive pressure ventilation.
However, experts argue about the practicality of the current definition of BPD, which fails to consider the newer modes of ventilation adopted in current practice. They also advocate for a more subjective grading of the severity of BPD that would justify interventions with higher risk–benefit profiles for babies predicted to develop more severe cases of BPD or more severe respiratory morbidity after discharge.8 The field is moving towards developing an updated definition of BPD based on prior definitions and current care practices. Recent research has shown that the best predictability of lung disease and neurodevelopmental injury at 18–21 months are oxygen requirement levels and/or respiratory support status at 40 weeks. Despite these considerations, there is still inconsistency among the definitions of BPD currently used for clinical trials and studies examining long-term outcomes.7
PATHOPHYSIOLOGY OF BRONCHOPULMONARY DYSPLASIA
BPD is the most common respiratory morbidity in preterm infants, affecting >10,000 babies in the USA each year.2 The aetiology of BPD is multifactorial and involves exposure to antenatal and/or postnatal factors that disrupt pulmonary development.9,10 In addition, the disease’s pathogenesis involves a complex interplay between environmental and host factors, including respiratory infections, genetic predispositions, intrauterine growth restriction, chorioamnionitis, oxidative stress, pulmonary fluid overload, and postnatal nutritional deficits.5 Both low GA and birth weight (BW) are commonly associated with higher rates of BPD. Patients who develop BPD are usually low BW infants (BW <2,500 g), very low BW (VLBW) infants (BW <1,500 g), or extremely low BW (ELBW) infants (BW <1,000 g). Hyperoxia, mechanical ventilation, nosocomial infection, male sex, patent ductus arteriosus, and congenital conditions are also risk factors for BPD. Some of these are known to induce lung injury, activation of inflammatory gene expression, and induction of the regulatory pathways involved in alveolar and vascular development.6
Understanding the pathogenesis and factors involved in BPD development is of significant importance in neonatal care, not only to design better preventative strategies for the disease but also because BPD is often associated with several comorbidities, which range from severe complications to conditions that significantly increase the disease mortality risk; these include complications such as retinopathy of prematurity, impaired bone growth, intraventricular haemorrhage, periventricular leukomalacia, and neurodevelopmental abnormalities,11-15 as well as pulmonary hypertension of the newborn, which occurs most often in infants with severe BPD and increases the mortality risk by almost 40%.16-19
EXPERIMENTAL THERAPEUTICS: UPDATE ON ANIMAL STUDIES
Multiple studies involving large (sheep, lambs, pigs, and nonhuman primates) and small (rabbits, rats, and mice) animal models have been conducted to date.20 These have provided useful information on the disease’s pathogenesis and potential therapeutic targets.21,22 Although there is currently no standardised single model that fully captures disease pathogenesis, several animal models using a variety of lung injury stimuli through exposure to hyperoxia, activation of the inflammatory response, and/or mechanical ventilation appear to be widely accepted.23 These have been used to identify key players in the mechanisms leading to the disease’s development and progression, identify genetic and epigenetic contributions, and to test potential therapeutic targets.24-29
Effective experimental therapeutics reported to date in animal models range from maternal diet supplementation using lipids (e.g., omega-3/6 polyunsaturated fatty acids [PUFA w-3, PUFA w-6]), which confer prevention against hyperoxia-induced inflammation and pulmonary hypertension in a rat model,30 to nutritional interventions in mechanically ventilated newborn animals (Table 2).30-37 Among these experimental therapeutics, parenteral treatment with lipid emulsion preparations, including SMOFlipid® (Fresenius Kabi, Bad Homburg, Germany) and Intralipid® (Fresenius Kabi) (which vary in PUFA w-6 content), have been proposed in clinical studies. However, in a BPD model consisting of hyperoxia-exposed newborn guinea pigs, SMOFlipid administration induced greater oxidation and had pro-apoptotic effects, indicating the need for additional studies before its adoption in the clinical setting.31
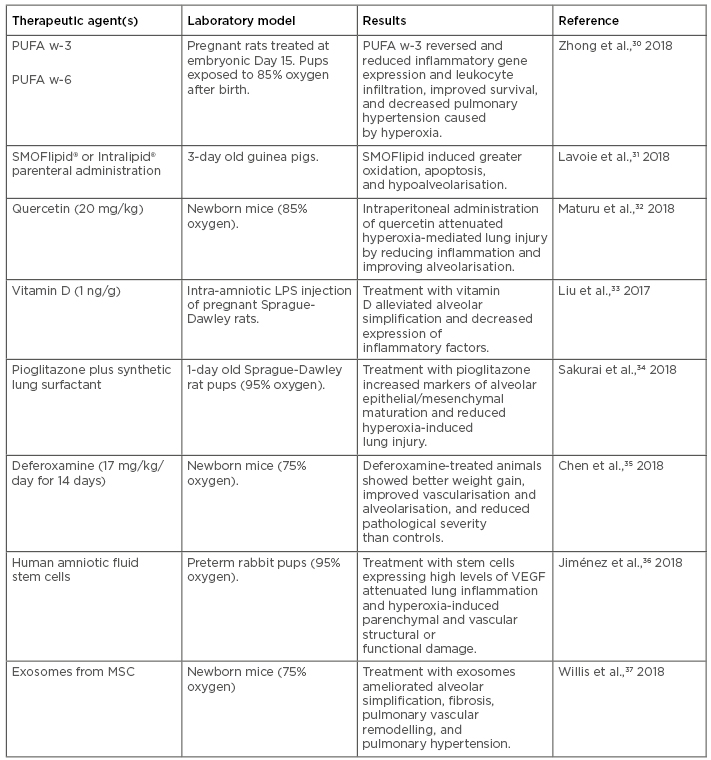
Table 2: Recent experimental therapeutics for bronchopulmonary dysplasia tested in animal models.
LPS: lipopolysaccharide; MSC: mesenchymal stem/stromal cells; PUFA: polyunsaturated fatty acids; VEGF: vascular endothelial growth factor.
Regarding natural products such as flavonols, which are compounds derived from fruits and vegetables that possess anti-oxidant and anti-inflammatory properties, a recent study of postnatal intraperitoneal administration of the flavonoid quercetin in mice showed it conferred protection to hyperoxia-induced lung injury via reduction of inflammation and oxidative stress marker expression, and improved alveolarisation outcomes, indicating the potential therapeutic value of flavonoids in BPD.32
Treatment with vitamin D has also proven effective at preventing BPD in a rat model.33 A study using intra-amniotic lipopolysaccharide (LPS) administration in pregnant rats, which is known to induce BPD in pups, showed that treatment with vitamin D for 7 days starting at postnatal Day 0 alleviated structural alveolar lung simplification induced by BPD and suppressed LPS-induced lung and spleen inflammatory gene expression, particularly IFN-γ.33 This study also explored the association of maternal vitamin D exposure during pregnancy and neonatal IFN-γ levels in a prospective birth cohort, finding a similar trend to that observed in the animal model.
Regarding pharmacological treatments, aerosolised administration of the iron-chelating drug deferoxamine for 2 weeks resulted in improved weight gain, reduced BPD severity, increased hypoxia-inducible factor-1α expression, and activation of vascular endothelial growth factor (VEGF)-induced angiogenesis in a mouse model of hyperoxia-induced BPD.35 Similarly, a combination of the peroxisome proliferator-activated receptor-gamma (PPAR-γ) agonist pioglitazone with a synthetic lung surfactant mix effectively accelerated lung maturation and prevented hyperoxia-induced lung injury when nebulised in a mouse model of hyperoxia-induced BPD.34 Experimental studies have also revealed that rosiglitazone (PPAR-γ) treatment can enhance pulmonary vascular development, restore alveolar function, and combat pulmonary hypertension by affecting VEGF and its receptor. PPAR belong to the superfamily of nuclear receptors; these are ligand-regulated transcription factors that control gene expression by binding to specific response elements within promoters and regulate energy homeostasis, lipid and glucose metabolism, and inflammation. PPAR-γ is predominantly found in white adipose tissue and has a role in storing triglycerides and fatty acids; however, the role of PPAR in pulmonary hypertension and alveolar function is still not known.
Finally, more recent experimental strategies to prevent and treat BPD have incorporated cell therapeutic approaches, including administration of mesenchymal stem cells (MSC), and cell-free therapeutics, such as the use of extracellular vesicles, including exosomes.37-44 For example, a recent study of postnatal intraperitoneal injection of amniotic fluid stem cells in a rabbit model of hyperoxia-induced BPD showed attenuated inflammation and improvement in parenchymal and vascular structure and function, particularly when using cells in which VEGF expression was upregulated.36 A more recent study used exosomes purified from human MSC culture media in a murine model of BPD and showed significant improvement in the lung inflammatory phenotype and pulmonary hypertension.37 Taken together, these recent studies indicate that BPD management and prevention could potentially be achieved by a combination of nutritional, pharmacological, and cellular therapeutic approaches, with promising results. Future work on the characterisation of mechanisms associated with the observed outcomes in animal models will help in the design of new clinical studies assessing the effectiveness of these experimental agents and/or nutritional management strategies in preventing or ameliorating BPD symptoms.
EXPERIMENTAL THERAPEUTICS: RECENT CLINICAL STUDIES
In the past few years, a number of prospective and retrospective clinical studies aimed to prevent and treat BPD have been conducted using a variety of agents and ventilation management approaches. A summary of the most recent studies using these compounds and the most significant outcomes are shown in Table 3.45-59 These studies have provided useful information on the effectiveness and pharmacological properties of various therapeutic agents, as well as other treatments for sepsis, inflammation, and concurrent infection in BPD patients.57,58,60 Of the most frequently used therapeutic agents for BPD management, the two most common compounds are caffeine and vitamin A. These are used either alone or in combination with ventilation management strategies, inhaled nitric oxide administration, systemic corticosteroid treatment, and other nutritional interventions.
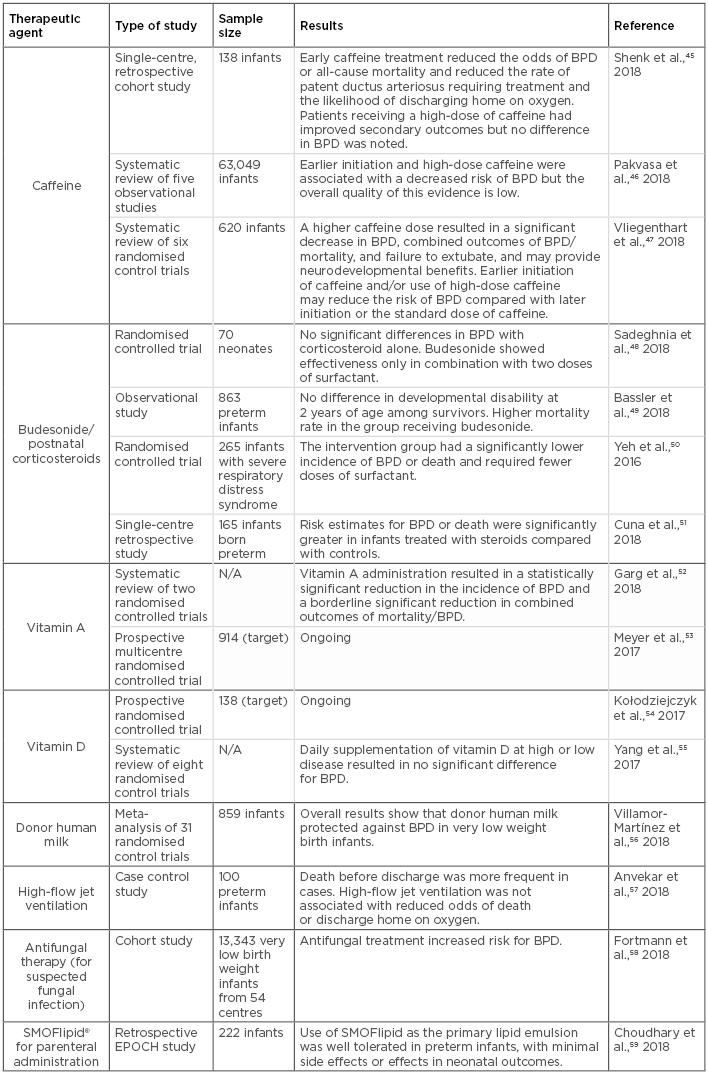
Table 3: Clinical studies assessing potential therapeutic agents for bronchopulmonary dysplasia in neonates.
BPD: bronchopulmonary dysplasia; N/A: not applicable.
Caffeine is one of the most widely used drugs in the neonatal intensive care unit. Initially used as a potent respiratory stimulant to treat apnoea of premature infants, as well as intermittent hypoxaemia and complications of extubation in mechanically ventilated infants, caffeine has been used to treat BPD and patent ductus arteriosus for many years. In addition, multiple studies have shown that its use has a positive effect on BPD outcomes due to its anti-inflammatory and diuretic properties, resulting in a shorter duration of mechanical ventilation.61 However, evidence regarding the timing of caffeine treatment initiation is unclear, since several observational studies have reported differential outcomes resulting from early and late caffeine use.46 For example, early caffeine use is considered a controversial practice because the benefits are limited to observational data, and associations of cerebellar injury with high-dose caffeine started early after birth have been reported.62 In contrast, late caffeine use has been associated with refractory respiratory failure, increased duration of mechanical ventilation, and increased requirement of postnatal steroids.45 Common current practice involves starting caffeine therapy in preterm infants early, often before the second day after birth.47 This practice may reduce extubation failure and the incidence of adverse neurodevelopmental outcomes.63 However, the dosage should be considered carefully, as caffeine use outside of the therapeutic range has also resulted in proinflammatory pulmonary outcomes.47 In the absence of concrete evidence, the optimal timing and dosage of caffeine administration for maximal benefit remain unclear.
Either alone or in combination with caffeine treatment, vitamin A supplementation has also been used as a preventative practice against BPD due to its proposed role in lung maturation. Its use is also often justified because preterm infants are particularly predisposed to vitamin A deficiency, and evidence from in vitro studies has showed vitamin A can promote DNA repair and alveolarisation. However, despite initially promising results, vitamin A supplementation is not currently widely implemented due to recent concerns regarding sepsis, discomfort (from repeated intramuscular administration), and necrotising enterocolitis (in small-scale studies).64,65 Although the most recent Cochrane review on the topic showed a modest reduction in the risk of BPD in VLBW infants,52 evidence from a nationwide decline in vitamin A supplementation during a recent drug shortage showed that BPD rates remained stable, thus leading investigators to question its efficacy.66 In this regard, the ongoing multicentre trial NeoVitaA53 aims to assess the ability of high-dose oral vitamin A supplementation to reduce BPD rates in infants weighing <1,000 g at birth and treated for 28 days. The results from these trials will hopefully provide clarification on the use of oral vitamin A as an effective treatment to prevent BPD in small, preterm infants.
Regarding postnatal corticosteroid therapy, including dexamethasone and budesonide, the evidence remains controversial. While recent clinical trials have shown that when administered systemically corticosteroid use results in significant improvements in short-term lung function and a reduction of BPD in infants at increased risk,51 these results have only been repeated in combination with surfactant therapy in a separate cohort,50 or showed no significant improvement in other studies.48,49 Moreover, systemic corticosteroid administration has also been associated with serious short and long-term adverse effects,67 making this practice less appealing due to safety concerns. Therefore, additional multicentre randomised controlled trials with proper follow-up studies are needed to further validate the benefits of prophylactic or therapeutic corticosteroid use in BPD patients.
Nutritional supplementation therapies have also been implemented in BPD clinical trials. In a recent review and meta-analysis of 31 randomised controlled trials and observational studies, investigators found that administration of donor human milk conferred protection against BPD in VLBW preterm infants.56 This meta-analysis had several limitations, including the observational nature of many of the studies included; that the studies displayed substantial heterogeneity in duration of intervention, timing of initiation, and population; and that none of the included randomised controlled trials were powered to detect the effects of donor human milk (HM) on BPD. In a prospective study of very preterm infants who received HM-derived cream, it was found that those receiving a HM-derived cream supplement were discharged earlier than the control group and, among them, infants with BPD benefitted the most.68 In another cohort study, it was found that increased doses of the mother’s own milk reduced the odds of BPD in VLBW infants.69 This notion has also been supported by a meta-analysis of multiple studies showing breast milk feeding in VLBW infants lowers the risk of BPD and associate comorbidities.70 On the other hand, vitamin D supplementation remains controversial in its efficacy and dosage recommendation from various contradictory studies,54,55 although deficiency of this vitamin has been directly associated with increased severity of BPD in an African-American preterm cohort.71 Furthermore, ongoing clinical studies evaluating the effectiveness of lipid formulations, such as SMOFlipid, in parenteral nutrition for preterm infants have shown anti-inflammatory properties that can benefit BPD patients,59,72,73 although recent animal studies have indicated a potential pro-apoptotic and pro-oxidant effect of these preparations.31 Finally, as promising preclinical studies have shown effective therapeutic potential of various preparations of mesenchymal stem cells to reduce the risk and severity of BPD,38 at least three ongoing early Phase I clinical trials (NCT03378063,74 NCT01297205,75 and NCT0163247576) are exploring their effectiveness in the prevention and treatment of BPD in various prematurely born infant cohorts.
CONCLUSION AND FUTURE DIRECTIONS
The effective management and prevention of BPD continues to be challenging despite significant advances in neonatal care, and the information obtained from animal models and clinical studies remains controversial. In addition, the efficacy of currently available therapeutic and preventative strategies varies depending on the populations studied and disease stage. Work conducted in animal models shows promising results on the potential use of pharmacological interventions, ventilation and nutritional management, and stem cell therapeutics to prevent and treat BPD. These studies have also provided information that could help identify the mechanisms behind the disease development and progression. As the results from clinical studies on the most commonly used BPD therapeutics remain contradictory and ambiguous, additional randomised controlled trials, together with longitudinal evaluation of potential beneficial or negative effects, are essential to optimise disease management and preventative strategies. As the rates of BPD continue to increase due to increased survival of VLBW preterm infants, it is imperative that we continue to investigate the mechanisms of BPD pathogenesis as well as alternative approaches for its treatment and prevention.