Abstract
Nanotechnology has become an important approach to improving the diagnosis and treatment of cancer; advances in this area have made it possible to use various materials to detect cancers in the early stages. Materials at the nanoscale have unique physical, optical, and electrical properties that are useful for cancer detection. Nanoparticles, alongside the discovery of several biomarkers, made it possible to reduce the detection limit of cancer biomarkers and this breakthrough provided the possibility of new methodologies for diagnosis with simple and non-invasive approaches. Haematological malignancies such as leukaemia and lymphoma represent a specific class of cancer that attract special attention in this area of diagnoses. The aim of this review is to elucidate the applications of nanotechnology for these types of cancer and the potentialities of nanotechnology for the diagnosis of haematological malignancies. When combined onto a single nanomaterial (an approach known as nanotheranostics), these platforms may revolutionise the way we tackle liquid tumours, as well as providing innovative tools for precision oncology, diagnostics, and follow-up therapy and disease management.
INTRODUCTION
Nanotechnology offers multiple solutions for cancer diagnostics and therapy, as it is capable of detecting even a single cancerous cell, as well as acting as a vehicle of drug delivery to the cell. Nanodiagnostic strategies are being developed to meet the requirements of clinical practice, providing increased sensitivity for early detection of disease biomarkers. Nanoparticles play a central role in the development of the new generation of platforms for cancer biomarkers detection, which is essential for precise treatment regimes. The versatile structural and functional properties of nanoparticles, which are incomparable to bulk materials, pave the way for the development of rapid, specific, and sensitive diagnostics, opening the door for decentralised assessments and/or ambulatory follow-ups.1
Despite their significant impact, haematological disorders have been rather neglected where nanotechnology platforms are concerned; the majority of these techniques have been directed at solid tumours. Nevertheless, haematological disorders are very good candidates for this approach, since the molecular basis of each sub-group of patients is well-defined by typified molecular alteration that may easily be integrated to develop suitable diagnostic schemes.Additionally, when retrieving a patient’s blood sample (liquid biopsy), one is gathering information on the patient’s full tumour profile via a less invasive routine.
BASICS OF LIQUID TUMOURS
Haematological malignancies comprise several different disorders, such as leukaemia, lymphoma, and myeloma, which affect the bone marrow, lymphatic system, and blood cells, respectively. In fact, haematological malignancies are the most frequent cancer type in children and young adults.2 Haematopoietic differentiation provides multiple occasions for mutations and other disruptive events to occur, resulting in distinct tumour subtypes and varying clinical presentations.3 The tremendous heterogeneity of haematopoietic and lymphoid tumours presents unique challenges for diagnosis and treatment.4-6 In the following section, we detail some of these differences and highlight the relevant biomarkers of disease.
Leukaemia
Leukaemia is a clonal disorder originating in the bone marrow during haematopoiesis, characterised by unregulated proliferation of poorly differentiated white blood cells (blasts). Disease classification is based on the affected cell type (myeloid or lymphoid) and the degree of cell proliferation (acute or chronic).3 Acute myeloid leukaemia (AML) is most common in adults, while acute lymphocytic leukaemia (ALL) is more prevalent in children.2 Chronic myeloid leukaemia (CML) is a myeloproliferative disorder with an annual incidence of 1–2 cases per 100,000 adults, accounting for 15–20% of newly diagnosed adult cases of leukaemia.2,7
Lymphoma
Lymphoma originates in the lymph nodes, where the lymphoid lineage of haematopoiesis differentiates into B, T, or natural killer cells. Several abnormal events may be observed in lymphoma, such as extensive cell proliferation, somatic mutations, and antibody class switching, that ultimately impair the immune system, particularly its adaptive capabilities.8 It is the most common haematological cancer in more developed countries, mostly associated with the formation of aberrant B cells.8 Lymphomas and lymphoid leukaemia, both included in the lymphoid neoplasm category, are closely related; in fact, chronic lymphocytic leukaemia (CLL) and small lymphocytic lymphoma (SLL) are essentially the same disease, the only difference being the location of the primary occurrence of cancer. In CLL, a significant number of the anomalous lymphocytes are found in the blood, bone marrow, and lymphoid tissue; in SLL, the bulk of the disease is in the lymph nodes, bone marrow, and other lymphoid tissues, but is rarely detected in peripheral blood. Both conditions are tackled in the same way, using immunomodulating agents, monoclonal antibodies, or kinase inhibitors.9
Molecular Characterisation
The laboratory diagnosis for haematological malignancies usually follows clinical depiction and includes morphological and immunophenotypic (via flow cytometry or immunocytochemistry) characterisation.10 Genetics play an important role for the classification of these disorders, since they originate from common chromosomal abnormalities that cause fusion of genes, a critical step in cancer progression (Table 1). Moreover, identification of mutations in specific genes allow for risk stratification, from poor to favourable prognosis.11 These distinguishing genomic alterations and associated chimeric genes are easily detectable via cytogenetics (karyotype or fluorescence in situ hybridisation [FISH]). At the molecular level, quantification of fusion gene expression via polymerase chain reaction (PCR) is mandatory and point mutations are typically identified via Sanger sequencing.12 In haematopathology, the use of molecular markers is key to integrated diagnostics, prognostics, and therapeutics. CML is a prime example of how haematological diseases have greatly benefited from the advance of cytogenetic and molecular methodologies. It was progress in this field that led, for the first time, to the identification of a unique chromosomal abnormality that was directly related to cancer onset: t(9;22)(q34;q11), forming what is known as the Philadelphia chromosome (Ph), and the associated BCR-ABL1 fusion gene, providing a specific target for disease treatment.1 In fact, tyrosine kinase inhibitors target the fusion protein encoded by BCR-ABL1 so as to block the dysregulated kinase activity.
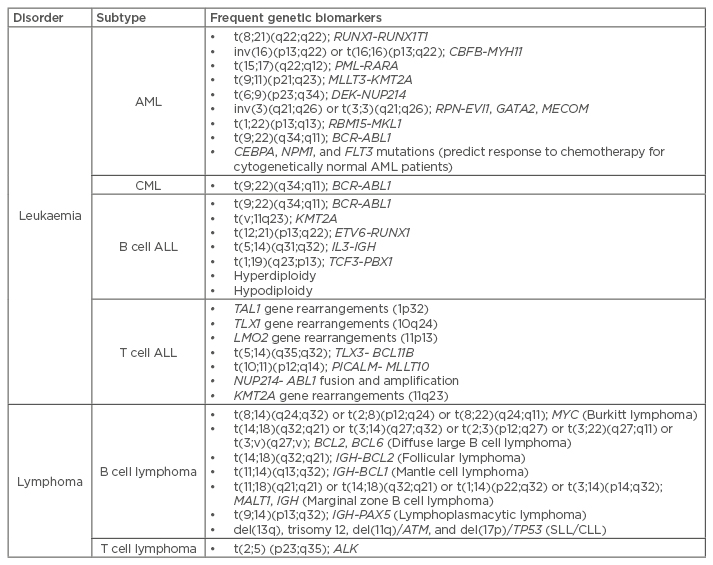
Table 1: Common genetic abnormalities associated to haematological disorders.11,13
ALL: acute lymphocytic leukaemia; AML: acute myeloid leukaemia; CLL: chronic lymphocytic leukaemia; CML: chronic myeloid leukaemia; SLL: small lymphocytic leukaemia.
LIQUID BIOPSIES IN LEUKAEMIA AND LYMPHOMA
The diagnosis and molecular management of cancer, including follow-up and companion diagnostics, are mainly performed on tissue biopsies that provide material for histological characterisation of the tumour and genetic assessment. These biopsies then facilitate the characterisation of the cancer subtype, indicating the appropriate therapy and, eventually, predicting therapeutic response.14,15 When one refers to solid tumours, biopsies are only a small portion of the neoplasm, not representing the existing heterogeneity. Usually performed at a specific time-point, the tumour dynamics and mutation patterns are neither assessed nor represent the whole cancer. Taking multiple biopsies from patients is not feasible, mainly due to the invasiveness of the procedure, the considerable risk, and, above all, the tremendous discomfort suffered by the patient, along with the considerable costs.
In myeloid and/or lymphoid neoplasms, conventional diagnostics consist of blood counts and biomarker evaluation on peripheral blood cells, complemented by morphologic examination of tumour tissues, which includes a collection of a substantial core biopsy of the bone marrow using a fine needle, or an excisional biopsy of lymph node or extranodal sites.16 Follow-up relies on peripheral blood assessments every 3 months for morphological, cytogenetic, or molecular marker evaluation. Since there is a good correlation between blood and core biopsies in haematological disorders, whether bone marrow or lymph node, biopsies are still necessary.17,18
The discovery of circulation tumour cells (CTC) and circulating cell-free DNA (cfDNA) presents clinicians with the opportunity for non-invasive disease monitoring techniques, i.e., liquid biopsies.15,19,20 Nowadays, from a peripheral blood sample it is possible to screen CTC, DNA, and other circulating biomarkers, such as microRNA (miRNA) and vesicles, capable of retrieving valuable information before and during treatment.20-22
Circulation Tumour Cells as Biomarkers
CTC can provide valuable information about tumour composition, invasiveness, drug susceptibility, and resistance to therapy, which in turn, through its isolation and further molecular and cellular characterisation, can allow a personalised approach to treatment, guiding chemotherapy and targeted therapy approaches.23,24 CTC can be passively or actively released to the bloodstream from the tumour site; however, most of them are accidental, since they are pushed by external forces (e.g., tumour growth). Dead tumour cells may passively release DNA or RNA into the circulation, or do so actively when cells release nucleic acids spontaneously.21
Circulating-Free DNA as a Biomarker
cfDNA and lymphocyte DNA are two types of DNA present in blood.25 cfDNA comes from rapidly dividing tissues, such as bone marrow, intestinal epithelium, or the fetus during pregnancy, and serum specimens from lymphoid neoplasms patients feature higher levels of cfDNA.20,25 Only a small percentage of cfDNA actually corresponds to circulating tumour DNA (ctDNA), which is less stable and displays different cytosine and guanine composition than that of DNA from non-tumour cells.20 Since ctDNA levels are higher in the later stages of disease, screening cfDNA allows a correlation to be made between molecular heterogeneity and therapy response.20,26 For example, in one study, at diagnosis, lymphoma patients had a cfDNA median concentration of 24.1 ng/mL, twice as much as the healthy controls; after 2 years of follow-up within patients with complete response to therapy, nearly 90% showed a significant decrease in cfDNA levels.20 Assessing ctDNA levels to monitor cancer rather than solely for diagnosis has been proposed, where the search for the characteristic BCL2-IGH translocation also allows identification of minimal residual follicular lymphoma.24 Another example is monitorisation of the diffuse large B cell lymphoma disease status, where ctDNA screening identified risk of recurrence before clinical evidence, resulting in further reduced disease burden at relapse.27 Additionally, cfDNA is detectable in 96% of diffuse large B cell lymphoma patients and may be used to detect somatic variants in this type of tumour.28
Exosomes and Vesicles as Biomarkers
Exosomes play an important role in cell communication, being able to induce transformations in receptor cells, including activation, proliferation, differentiation, or apoptosis.29,30 Exosomes are small vesicles (30–100 nm) that transport proteins, messenger RNA (mRNA), and miRNA, whose overall composition depends on the cell type or tissue that they are released from, as well as the cell’s physiological condition.31 In addition to CTC and cfDNA, since tumour cells release exosomes at a higher rate, exosomes present growing interest in oncology.20 Cancer-derived exosomes present characteristic cargo that may provide not only information about the pathophysiological status of cancer patients, but also disease detection and monitoring. Particularly, their RNA content displays several advantages when compared to DNA, such as information on coding mRNA that might indicate which particular genes were transcribed and expressed as proteins; or the analysis of small noncoding miRNA, indicating the presence of particular types of cancer. Although there is a high ribonuclease (RNase) activity in blood, which would render free-RNA useless, encapsulation of this RNA into exosomes allows their retrieval and use as valuable biomarkers.32
Despite considerable knowledge of the exosome role in solid tumours, their role in haematological tumours is not well-studied.30,33 As for cfDNA levels, higher levels of exosomes are found in cancer patients. For instance, concentration of exosomes in plasma increased along with AML development, and decreased after a conventional therapy regimen with parallel reduction of blasts in bone marrow.30,34 Exosome reduction is also associated with a quantitative change of exosome-derived proteins. In this case, studies propose that the tumour growth factor β1 levels were higher upon AML diagnosis compared to normal controls, and these levels were significantly reduced following chemotherapy.33 Exosome miRNA has also been proposed as an early biomarker for AML.30 In CML-derived exosomes, a specific expression pattern of miRNA was unveiled, which may be further explored for future use as disease biomarkers.33 In fact, higher concentrations of exosomes are found in the sera of AML, CML, and CLL patients, with abundant expression of surface proteins according to their cell of origin that is rarely observed in exosomes of healthy individuals. Together, this supports the use of exosomes as cancer biomarkers.
Liquid biopsies are associated with circulating material, mainly CTC, nucleic acids, and exosomes. However, this is dependent on which liquid tumours are concerned, since a direct sample of tumour cells may be retrieved from a peripheral blood collection. As a result, besides the usual counting of leukocytes, haemoglobin, lymphocytes, and platelet levels, new prognostic markers should be addressed as far as disease outcome and clinical decision-making are concerned.35 This also allows for detection of molecular markers, such as point mutations and chromosomic aberrations related with each lymphoid neoplasm subtype. Gomes et al.35 observed significant changes for phospho-p38 and Mcl-1 proteins in CLL patients when compared to the control group. Mcl-1 protein, an anti-apoptotic protein of the Bcl-2 family, was associated with malignant cell survival, worse prognosis, and chemoresistance in CLL. Low levels of phospho-p38 also endorse apoptosis resistance, since p38 is a protein of MAPK family with a pro-apoptotic role. Circulating miRNA in the plasma of AML patients showed upregulation of let-7b and miR-523 and downregulation of let-7d, miR-150, miR-339, and miR-342, independent of patient sex.36
NANOPARTICLE APPLICATIONS IN LIQUID TUMOURS
The progressive field of nanotechnology has had a tremendous impact on biomedicine, now known as nanomedicine. Cancer nanomedicine has raised the stakes towards the improvement of detection limitations for cancer biomarkers, but therapeutics could also benefit from these new strategies, which are more target-driven than traditional chemotherapeutics. Among the plethora of different nanoscale devices applied to cancer diagnostics and therapeutics, here we will focus on nanoparticles, which are synthesised in the range of 1–100 nm and feature unique optical, electronic, and catalytic properties, very different from those of the bulk material.37,38 Concerning liquid tumour diagnostics, different approaches using nanoparticles have been proposed towards better diagnostics, imaging, and treatment.
Nanodiagnostics in Liquid Tumours
Traditionally, leukaemia and lymphoma cells are detected through morphological analysis, immunohistochemistry, microarray of antibodies, flow cytometry, FISH, PCR, and DNA sequencing. These techniques are costly and time consuming, requiring sophisticated instrumentation and multi-step processing, and are not suitable for rapid routine testing.39 To overcome these shortfalls, several efforts have been directed at using nanoparticles for liquid tumour testing and assessment. These platforms may be used to detect biomarkers at lower concentrations more quickly than a non-invasive approach. One of the major problems in diagnostics in lymphoid neoplasms is the limit of detection of immature white blood cells, because their abundance is very low at such an early stage. Since these cancer types are extremely common and aggressive, an effective treatment largely depends on the accuracy and sensitivity of diagnosis.40 Signal amplification coupled with nanoparticles can be a viable approach for an earlier detection, leading to fluorescent quantum dots and nanometre semi-conductors, which have superior fluorescent features, being applied in the diagnostic field. In addition to fluorescent enhancement, metal nanoparticles feature other unique physicochemical properties, including their localised surface plasmon resonance, as well as photoluminescence or superparamagnetic properties, which turn them into extremely good candidates for imaging and diagnostics.41 Some of these platforms are close to the clinics, but still need to provide convincing performance data in the diagnostics lab (Table 2).
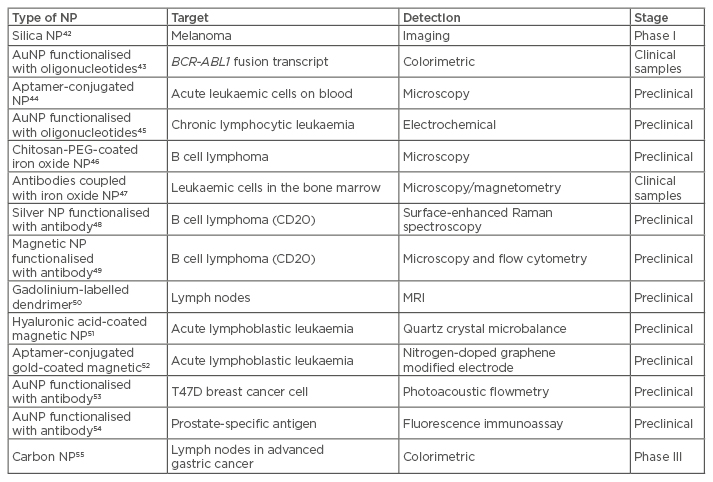
Table 2: Nanoparticles for molecular diagnostics in liquid tumours.
AuNP: gold nanoparticles; MRI: magnetic resonance imaging; NP: nanoparticles; PEG: polyethylene glycol.
For acute leukaemia diagnostics, an anti-leukaemia thiolated aptamer (such as sgc8c) is used to specifically recognise protein tyrosine kinase 7, an overexpressed transmembrane receptor in human T cell ALL cells.39,40,52 Aptamers feature a high affinity and selectivity towards their targets, as well as being extremely sensitive, allowing an accurate detection of cancer cells. Aptamers are also inexpensive, easier to produce and modify, have more stability, and less immunogenicity or toxicity than antibodies.40,52
Concerning CML, since it is associated to a unique chromosomal abnormality, the Ph chromosome and the corresponding BCR-ABL1 gene, this specific target is used as a diagnostic approach. Between the existent strategies using nanoparticles, we can only find examples with gold nanoparticles.43,56 Both examples are based on the optical properties of gold nanoparticles. The former relies on a colorimetric differentiation upon salt-induced aggregation, where a positive test reveals a red colour; whereas in a negative test, the gold nanoparticles aggregate, yielding a blue colour solution. Gold nanoparticles are functionalised with oligonucleotides that specifically hybridise to the target, in this case the BCR-ABL1 gene.43,57 Gold nanoparticles are also known to modulate the fluorescence of nearby fluorophores, which have been used for a multitude of sensing approaches for DNA and RNA. One example is the BioCode approach, a spectral coding approach using fluorescence energy transfer for the distinction of e14a2 and e13a2 transcript fusions in CML. In this approach, a complementary sequence to the loop portion of hairpin-functionalised gold nanoparticles allowed for fluorophore emission. Then a second (revealing) fluorescent oligo hybridises to the palindrome part of the hairpin promoting fluorescence energy transfer between the fluorophores and a specific spectral signature.1
The remaining targets for both leukaemia and lymphoma diagnostics are antigens, such as CD20, which are overexpressed by malignant B cells.58 In this case, CD20 antibody (rituximab) has been applied to lymphoma treatment, but is also suitable for lymphoid neoplasms diagnosis.49 Besides CD20, CD45 and CD19 are the two most common surface proteins expressed by B cells, and are used in diagnostic immunophenotyping.59 Based on these molecular differences, it is possible to diagnose, image, and isolate malignant B cells via gold nanoparticles and surface-enhanced Raman scattering, magnetic nanoparticles, and polymeric-fluorophore-labelled nanoparticles, respectively.49,59,60
FUTURE PERSPECTIVE ON HAEMATOLOGICAL MALIGNANCIES
Many features of nanoparticles have been described in this review that are particularly attractive for biomarker detection. This versatility makes them suitable candidates for nanotheranostics, a central approach in precision oncology, since it allows the integration of diagnostics and therapeutics onto a single material. When designing nanotheranostics for haematological malignancies, one should take into consideration the following parameters: a) choice of the most suitable nanocarrier for effective delivery and cell internalisation; b) the effector molecule, usually conventional drugs or nucleic acids; c) ensure controlled release of effector molecule; d) an imaging component that allows for real-time monitoring of the nanoconjugate location and effect on targeted aberrant cells; e) an active targeting agent, typically a cell-surface marker or an oligonucleotide that maximises specificity of the vector and minimises damages to healthy cells, whether in peripheral blood or when targeting the bone marrow or lymphoid tissues.
Because most of these liquid tumours are closely linked to specific molecular events, novel nanoparticle-based detection schemes could be easily implemented both for diagnostics and, above all, for follow-up therapy and disease management. Several nanoformulations have also been proposed for improving therapeutics by means of smart nanoparticle designs capable of searching for and destroying malignant cells.